Written byAaron Gronstal


Many factors affect the habitability of Earth, but one of the most influential factors is our star, the Sun. The Sun bathes our planet in radiation and is the primary source of energy for Earth’s biosphere. Primary producers, from microbes to plants, convert radiation from the Sun into food. But there are two sides of the coin when it comes to radiation. It provides energy for life, but it can also be incredibly damaging to biology.
Radiation can be thought of in two categories, non-ionizing and ionizing. These two categories of radiation sit at either end of a scale. In the middle of that scale is ultraviolet radiation (UV). Anyone who has spent time outside is familiar with UV radiation, especially if they’ve forgotten to wear sunscreen. Radiation with a wavelength below that of UV is non-ionizing, while wavelengths above are ionizing.
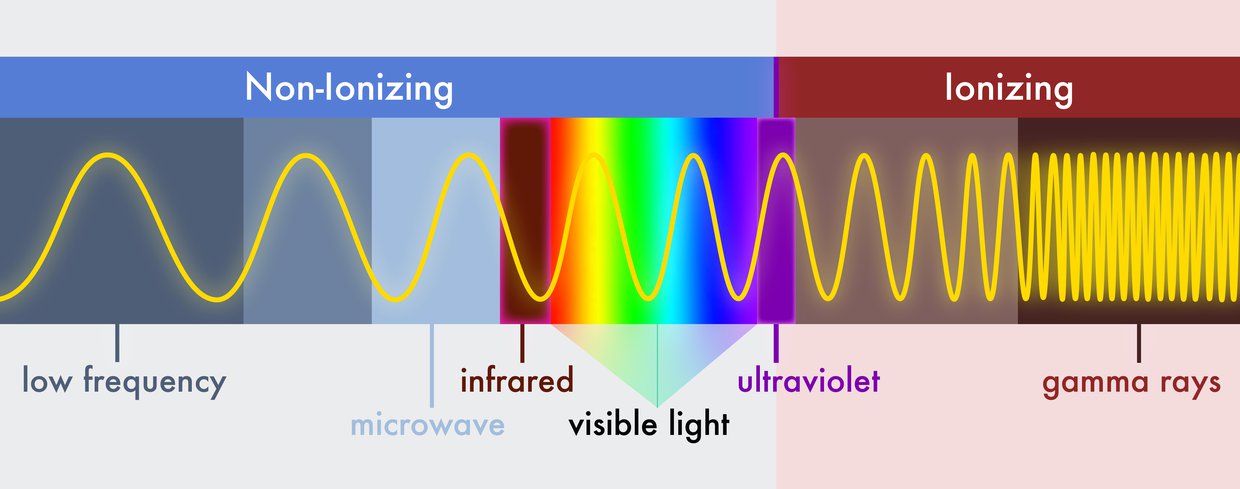
Non-ionizing and ionizing radiation are two broad categories that sit at either end of the scale above. Ionizing radiation includes the short-wavelength end of the spectrum. This type of high energy radiation can be very damaging for biology.Image credit: NASA Astrobiology.
Non-ionizing radiation includes things like radio waves and visible light, which do not break molecular bonds. Life on Earth is constantly exposed to non-ionizing radiation, most of which is harmless. However, intense exposure to non-ionizing radiation can cause harm in the form of overheating.
Once you get to UV radiation, real damage can start to occur… damage far worse than sunburnt skin. Wavelengths above UV radiation bring you into the realm of ionizing radiation (such as gamma radiation, x-rays, etc.), which can be very dangerous. If you’ve ever had an x-ray taken at the hospital you may remember having lead blankets placed on your body to shield areas from exposure. Ionizing radiation can break molecular bonds, causing serious damage to cells. High doses of ionizing radiation damage structures inside cells, such as DNA and proteins, and can cause mutations to occur. These mutations can result in all kinds of problems for cells, including cancer.
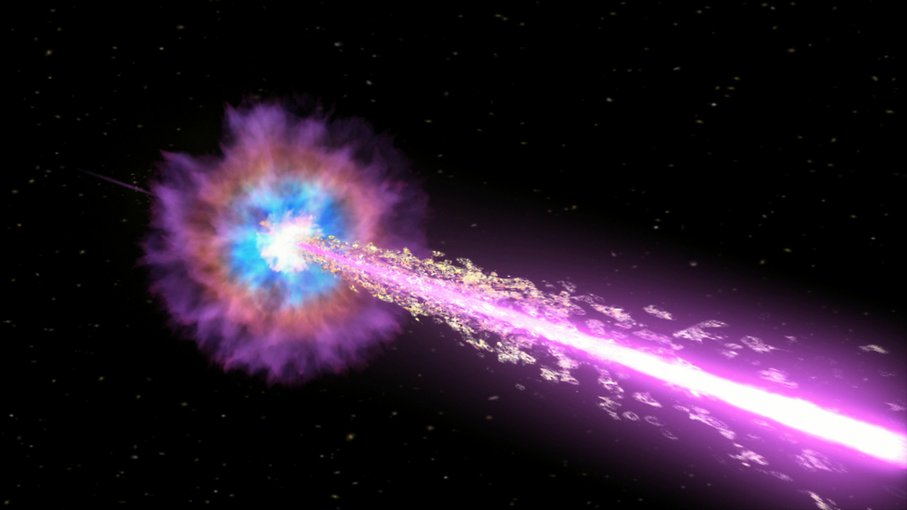
In this illustration, a black hole drives powerful jets of particles traveling near the speed of light. The jets pierce through a star, emitting X-rays and gamma rays as they stream into space.Image credit: NASA/Swift/Cruz deWilde.
There are many sources of radiation in addition to the Sun. Naturally occuring radiation comes from every star in the Universe as well as other objects like quasars and galactic centers. We are also constantly surrounded by sources of background radiation on Earth. This low-level radiation comes from things like radioactive elements in the Earth’s crust, or from human-made sources like microwaves, mobile phones, radios, and light bulbs (to name a few) [1, 2].
Space is also full of cosmic radiation, and life’s first line of defense against radiation in space is the Earth’s magnetosphere and atmosphere. The magnetosphere is like a giant bubble of magnetism that helps deflect radiation away from our planet. The magnetosphere also helps keep the Earth’s atmosphere from being stripped away into space (see the video above for more details). The atmosphere helps protect life at the Earth’s surface by absorbing harmful radiation. The thin ozone layer, for instance, absorbs most of the harmful UV radiation that Earth receives from the Sun [3].
Radiation Resistant Extremophiles
On Earth, astrobiologists study extremophile organisms known as radiation resistant (or radioresistant) organisms to better understand the mechanisms that life can use to protect itself from the ill effects of radiation. Organisms that are resistant to above-normal amounts of ionizing radiation can be found in all three domains of life (Bacteria, Archaea, and Eukarya). This means that this widespread ability likely evolved independently many times throughout history as organisms had to cope with changing environments that exposed them to high UV radiation, damaging chemicals, and limited access to water [4].
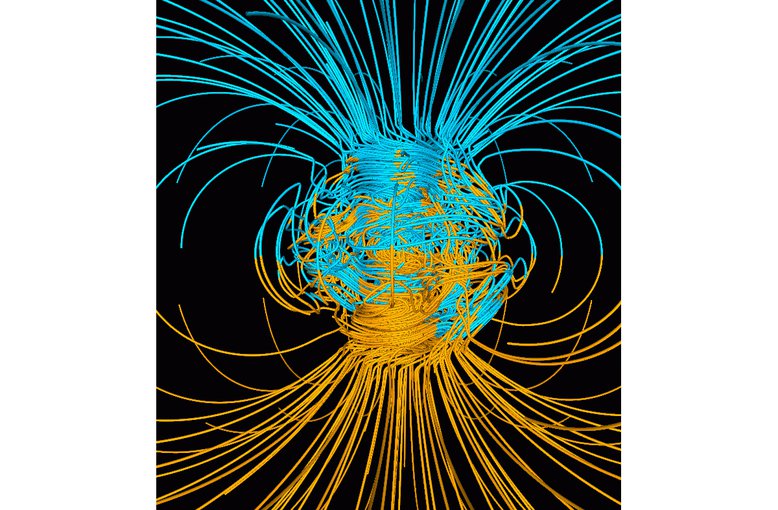
A supercomputer model of Earth’s magnetic field.Image credit: Gary A. Glatzmaier (UCSC)/NASA.
Earth’s magnetosphere and atmosphere help to shelter life from radiation, but there are still environments on Earth where radiation is high. In these places, life has developed strategies to survive. Some organisms can build protective structures to shield themselves, in a similar way that we use lead blankets to protect ourselves when getting an x-ray at the hospital. Other organisms live underground or inside rocks, and this provides physical shielding from the radiation outside. However, the real radioresistant extremophiles don’t just shelter themselves from radiation, they use it to their advantage.
After the Chernobyl disaster, scientists discovered radioresistant fungi growing in the destroyed nuclear reactor. They determined that these fungi (members of Eukarya) weren’t just surviving, they had developed ways of using the radiation from radioactivity as a source of energy. Because they use this energy source to make food and grow, these fungi are called ‘radiotrophic.’ There is still a lot to learn about radiotrophic fungi, but most are thought to gain energy by using a natural pigment called melanin in a process analogous to photosynthesis called radiosynthesis [5].
At Chernobyl, some 200 species of radiotrophic fungi containing melanin were identified. Similar fungi with melanin have also been found in environments with high levels of UV radiation at the top of mountains (where the thin atmosphere doesn’t provide as much protection for life). When these organisms were tested in the laboratory, they actually grew better in the presence of high radiation when compared to non-radiation environments [6].
Check out the set of trading cards featuring nine different extremophile groups at: https://astrobiology.nasa.gov/classroom-materials/tradingcards/Image credit: NASA Astrobiology.
For astrobiologists, however, one of the most studied (and most radioresistant) organisms on Earth is the bacterium Deinococcus radiodurans. This microbe can withstand exposure to extreme levels of ionizing radiation (including UV and gamma rays). In fact, these tiny organisms are capable of surviving doses of radiation 500 times higher than humans. On top of that, they can live in environments that are extremely dry, cold, and acidic.
Like any organism doused with ionizing radiation, Deinococcus radiodurans suffers multiple breaks in its DNA. For other organisms, this can be a catastrophic attack leading to cell death. Some ways in which Deinococcus radiodurans copes with radiation include keeping multiple copies of its DNA and isolating fragments caused by irradiation into a tightly condensed area so that they don’t diffuse through the cell. In addition, the enzymes used to repair DNA in Deinococcus radiodurans seem to be particularly efficient [7, 8].

A transmission electron micrograph shows Deinococcus radiodurans, a bacterium that is resistant to extreme levels of ionizing radiation and desiccation. Strains were isolated from sediments collected from under a nuclear waste storage tank.Image credit: Courtesy of Pacific Northwest National Laboratory.
Other radioresistant bacteria that have been identified include Kineococcus radiotolerans and thermophilic (able to withstand high temperature) Actinobacteria including Rubrobacter radiotolerans and Rubrobacter xylanophilus [8].
The domain Archaea includes a large number of extremophiles capable of surviving in conditions like high salinity, high temperature, extreme pH, and high radiation. Deep below the ocean around super hot hydrothermal vents, scientists discovered Thermococcus gammatolerans. As its name suggests, this thermophilic organism is able to tolerate high doses of gamma-irradiation [8]. One strategy this organism uses to stay safe is that it keeps the proteins used to repair DNA constantly active. This means that, rather than waiting for damage to occur, the cells are always ready and waiting to fix their DNA. Thermococcus gammatolerans also has special detoxification genes that help mitigate the ill effects of radiation damage [8].
Other radiation resistant archaea include Pyrococcus furiosus and Pyrococcus
abyssi. Like Thermococcus gammatolerans, these organisms are also hyperthermophiles (e.g. they really like heat). There seems to be a connection between thermophilic and radiation resistant organisms. This might be because high heat can cause damage that is similar to what happens in high radiation environments. Strategies for coping with one extreme environment might work in both.
Radiation in Space
Thanks to Earth’s atmosphere and magnetic field, the radiation environment on our planet is relatively mild compared to the vacuum of space and other locations in the Solar System. For instance, Mars doesn’t have a powerful, global magnetosphere or a thick atmosphere, so the surface of the red planet receives far more radiation than that of the Earth. To survive on Mars, cells might need adaptations similar to those we see in Deinococcus radiodurans.
Astrobiologists have used laboratory experiments to explore whether or not radioresistant organisms on Earth would be able to survive on planets like Mars. This helps scientists understand the kinds of strategies that life as we know it would need on Mars, and the types of biosignatures such life might leave behind. These experiments also help astrobiologists determine how much radiation is ‘too much’ for life as we know it. This is an important question when we’re trying to determine the conditions that make a world habitable.
Radiation studies are also an important part of NASA’s Planetary Protection research portfolio, which strives to make sure Earth microbes don’t travel along with space missions to planets that could be (or could have been) suitable for life. The worry is that an organism like Deinococcus radiodurans might hitch a ride to a place like Mars and contaminate the landing site, interfering with the search for uniquely martian biosignatures. Furthermore, if life were to exist on another world today, introducing organisms from Earth could be catastrophic for the local biosphere.
To test the radiation limits of life on Earth, and on other worlds, astrobiologists have built special laboratory equipment to simulate different radiation environments. These chambers are able to recreate some conditions that are found on worlds like Mars, Venus, and Europa. In these chambers, astrobiologists grow radiation resistant extremophiles to test their capabilities and limits [8].
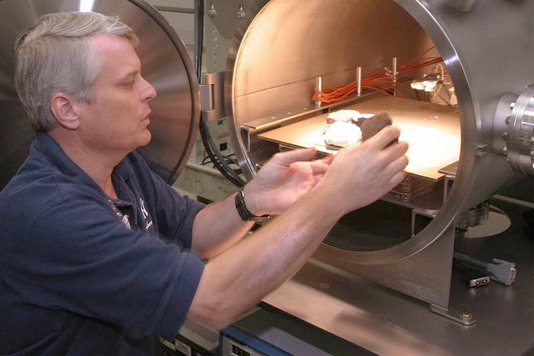
Andrew Schuerger placing samples in a Mars chamber at the University of Florida.Image credit: University of Florida.
What we know about the radiation environment at the surface of Mars comes from our long history of exploring the planet with robotic missions. For instance, the Curiosity Mars rover has used instruments to characterize a broad spectrum of primary and secondary high-energy radiation, as well as UV radiation. This information guides the development of future missions that will search for signs of past life and the planetary protection requirements needed to ensure their success. The study of extremophile survivability on Mars is becoming more and more important as NASA and international partners develop ‘search for life’ missions to the red planet, including Mars Sample Return.

A view from the “Kimberley” formation of Gale Crater on Mars taken by NASA’s Curiosity rover.Image credit: NASA/JPL-Caltech/MSSS.
The radiation limits of life as we know it can help determine the habitability of planets outside our solar system. Planetary systems orbiting stars different from our Sun can have radiation exposure levels much different than Earth, like the TRAPPIST-1 system, which orbits around an M Dwarf star that ejects violent radiation flares. Astrobiologists try to determine what a high-radiation environment around such stars could mean for the habitability of planets that orbit them. Understanding how life on Earth copes with high radiation is important in these studies because our planet is the only one we know of thus far that supports life.
Due to the protection of our atmosphere and magnetosphere, Earth has a favorable radiation environment compared to almost any other known location in the Solar System and beyond. Because the Universe is full of radiation, it could be one of the most important environmental factors in determining where and how life as we know it could gain a foothold on other worlds near and far.
Life in the Extreme:
Life in the Extreme: Radiation
Life in the Extreme: Surviving Beneath a Glacier, Part I
Life in the Extreme: Surviving Beneath a Glacier, Part II
Life in the Extreme: Terrestrial Hot Springs
Life in the Extreme: Polar Deserts
Life in the Extreme: Hydrothermal Vents
Related:
Life in the Extremes: Astrobiology Extremophile Trading Cards
Radioresistant Trading Card
Life at Low Pressure: Planetary Protection and the Habitability of Mars
Selection of Papers from Projects Supported by NASA Astrobiology: Radiation
Comparison of Martian Radiation Environment with International Space Station (NASA)
References:
(1) United States Environmental Protection Agency (2022) Radiation Sources and Doses. Available at: https://www.epa.gov/radiation/radiation-sources-and-doses
(2) NASA (2019) Why Space Radiation Matters. Available at: https://www.nasa.gov/analogs/nsrl/why-space-radiation-matters
(3) NASA (2023) NASA Ozone Watch. Available at: https://ozonewatch.gsfc.nasa.gov/
(4) Mironenko, NV., et. al. (2000) Intraspecific Variation in Gamma-Radiation Resistance and Genomic Structure in the Filamentous Fungus Alternaria alternata: A Case Study of Strains Inhabiting Chernobyl Reactor No. 4, Ecotoxicology and Environmental Safety, 45(2) DOI: DOI: 10.1016/j.mib.2008.09.013
(6) Dadachova, E., et. al. (2007) Ionizing Radiation Changes the Electronic Properties of Melanin and Enhances the Growth of Melanized Fungi, PLOS One, DOI: 10.1371/journal.pone.0000457
(7) Cox and Battista (2000) Deinococcus radiodurans — the consummate survivor. Nature Reviews Microbiology,3. DOI: 10.1038/nrmicro1264
(8) Jung, K.W., et. al. (2017) Microbial radiation-resistance mechanisms. Journal of Microbiology, 55(7). DOI: 10.1007/s12275-017-7242-5
(9) Schuerger, A.C. (2009) Chamber for Simulating Martian and Terrestrial Environments. NASA Tech Briefs. https://ntrs.nasa.gov/citations/20090029275