

The European Space Agency (ESA) has approved the ARIEL space mission—the world’s first dedicated exoplanet atmosphere sniffer— to fly in 2028.
ARIEL stands for the “Atmospheric Remote-sensing Infrared Exoplanet Large-Survey mission.” It is a space telescope that can detect which atoms and molecules are present in the atmosphere of an exoplanet.
The mission was selected as a medium class mission in the ESA Cosmic Vision program; the agency’s decadal plan for space missions that spans 2015 – 2025.
One of the central themes for Cosmic Vision is uncovering the conditions for planet formation and the origins of life. This has resulted in three dedicated exoplanet missions within the same decadal plan. ARIEL will join CHEOPS (in the small class mission category) and PLATO (another medium class mission) in studying worlds beyond our own sun.
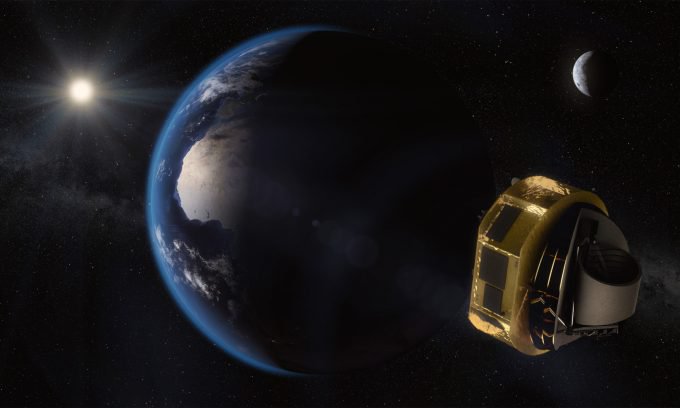
The Ariel space telescope will explore the atmospheres of exoplanets.Image credit: Artist impression, ESA.
Yet ARIEL is a different type of telescope from the other exoplanet-focused missions. To understand why, we need to examine what properties we can observe of these distance exo-worlds.
Exoplanet missions can be broadly divided into two types. The first type are the exoplanet hunter missions that search the skies for new worlds.
These are spacecraft and instruments such as NASA’s Kepler Space Telescope. Since it launched in 2009, Kepler has been an incredibly prolific planet hunter. The telescope has found thousands of planets, modeled their orbits and told us about the distribution of their sizes.
From Kepler, we have learnt that planet formation is common, that it can occur around stars far different from our own sun, and that these worlds can have a vast range of sizes and myriad of orbits quite unlike our own Solar System.
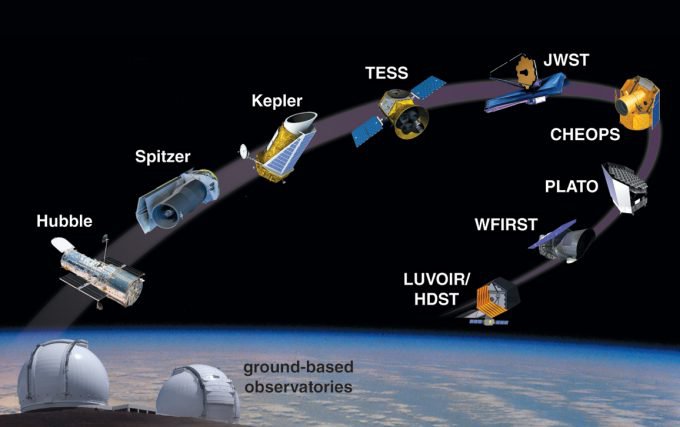
Current and future (or proposed) space missions with capacities to identify and characterize exoplanets.Image credit: NASA,ESA: T. Wynne/JPL, composited by Barbara Aulicino.
However, the information Kepler is able to provide about individual planets is very limited. The telescope monitors stars for the tiny drop in light as the planet crosses (or “transits”) the star’s surface. From this, astronomers can measure the radius of the planet and its orbital period but nothing about the planet’s surface conditions.
The result is a little like knowing the number of students and distribution of grades in a particular school, but having no idea if the student who sits in the third row actually likes math.
The second medium-class mission in the ESA Cosmic Vision program, PLATO, is also a planet hunter. Like Kepler, PLATO will search stars for the periodic light dip that indicates the presence of a planet.
However, the telescope will explore a much larger region of the sky than Kepler, with an emphasis on detecting rocky planets on Earth-like orbits that receive a similar amount of radiation as our own planet (the so-called habitable zone).
While PLATO will not be able to tell if these planets are actually Earth-like (and not just Earth-sized on similar orbits), it will tell us a lot more about the statistics of solar systems like our own. I’m also going to throw CHEOPS into this category at well.
Technically, CHEOPS is not a planet hunter as the telescope will search for the transit light dip of stars already known to host planets. These worlds have been detected by the wobble of the star due to the presence of the orbiting planet, a method known as the radial velocity or Doppler wobble technique.
This method provides the minimum mass of a planet, but the true mass depends on the angle of the planet’s orbit. If a transit could be detected, then both the radius and the orbit inclination would be known, providing a density for these worlds. Bulk density is a very useful measurement for differentiating between rocky super Earths and gaseous mini Neptunes, providing a huge boost information for planet formation theories.
However, CHEOPS still will not know anything about the composition or surface conditions of these planets.
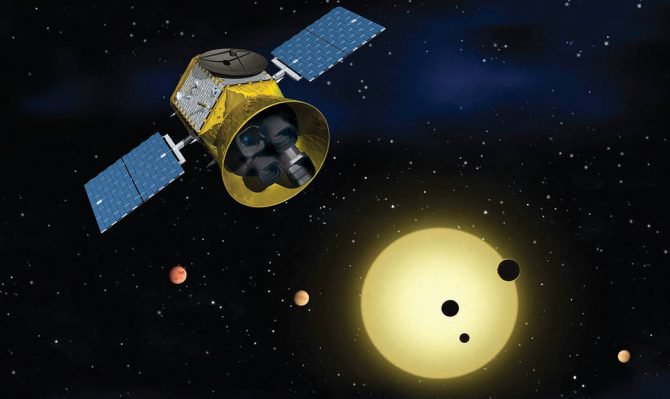
NASA’s Transiting Exoplanet Survey Satellite (TESS) is scheduled to launch next months.Image credit: Artist impression, NASA’s Goddard Space Flight Center.
By virtue of its imminent launch in the next month, the NASATESS mission is one of the most exciting new entries in the planet hunter class. TESS will sweep over the whole sky, a huge coverage that means the telescope must focus on planets orbiting bright stars at a swift click of about 10 days per orbit.
In a two-year survey of the solar neighborhood, TESS will monitor more than 200,000 stars for planetary transits. This first-ever space-borne all-sky transit survey will identify planets ranging from Earth-sized to gas giants, around a wide range of stellar types and orbital distances.
If we compare the planet hunter missions to our school analogy, Kepler studied the grades of one school, TESS is checking a single class in multiple schools and PLATO is aiming for a full educational census. CHEOPS is adding in extra scores to greatly extend the useful statistics.
One of the key goals of TESS is to identify good targets for atmospheric follow-up missions — a topic that brings us finally back to ARIEL.
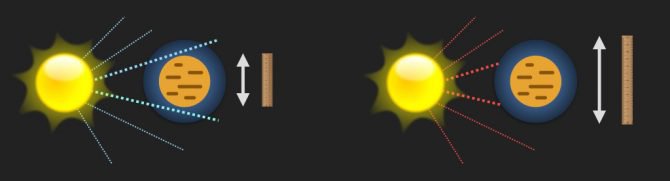
Transmission spectroscopy: Molecules in an exoplanet’s atmosphere absorb different wavelengths of light, causing the atmosphere to go from transparent (left) to opaque (right). The observed planet radius therefore depends on the wavelength being observed.Image credit: ESA.
In contrast to Kepler, PLATO and TESS, ARIEL is not trying to find new planets.Instead, the telescope belongs to the second type of mission which probes conditions on the planet itself.
ARIEL will look at starlight that is passing through the atmosphere of known transiting planets. Molecules in the atmosphere absorb different wavelengths of light, turning the gases surrounding the planet opaque at these wavelengths. This produces a change in the planet’s radius as its atmosphere switches from transparent to opaque.
By measuring this apparent size change for different wavelengths, ARIEL can decipher what gases must be in the planet’s atmosphere.
This technique is known as transmission spectroscopy, where the term spectroscopy refers to studying light split into its constituent wavelengths or spectrum. These molecules are the products of the planet’s composition, chemistry and —in the case of rocky planets— geological and potentially biological processes. This makes transmission spectroscopy a direct measure of what is going on within the planet.
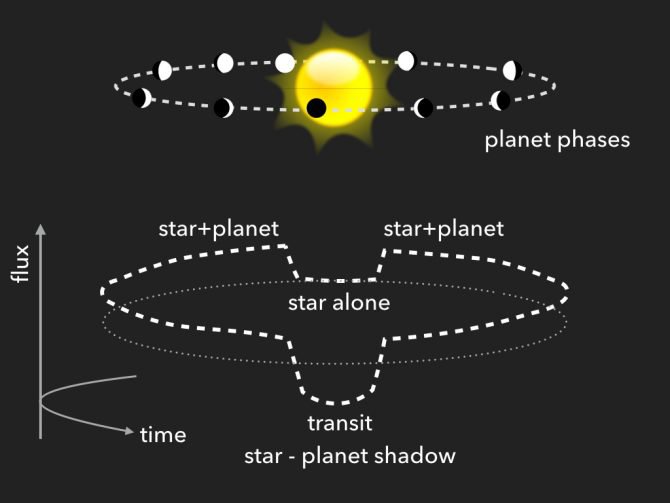
Eclipse spectrometry: As the planet is eclipsed by the star, a secondary dip in luminosity is observed. This corresponds to the planet’s own radiated and reflected light and can also reveal atmospheric details.Image credit: ESA.
In addition to exploring the light dip during the planet’s transit, ARIEL will also examine the tiny difference in radiation as the planet ducks behind the star.
We normally consider the planet as a dark object obscuring the star’s light during the transiting part of its orbit. However, the planet also emits radiation both due to its ownheat and reflected starlight. Just before the planet is eclipsed by the star, the observed luminosity peaks due to the combination of the star and fully illuminated planet.
When the planet moves behind the star and disappears from view, there is a sudden drop corresponding to the loss of the planet’s radiation. This fluctuation is more tiny than when the planet transits across the star, but its detection measures the planet’s own radiation.
The magnitude of this dip also depends on wavelength, as the structure and composition of the planet’s atmosphere will determine what wavelengths of radiation are being reflected and emitted.
Looking at the spectrum of this emitted (rather than transmitted) light gives ARIEL a second handle on planet conditions. The planet’s disappearance behind the star is known as the secondary or planetary eclipse giving this technique the name eclipse spectroscopy.
As well as studying the components of light as the planet and star eclipse one another, ARIEL will also monitor the luminosity of the planet during its whole orbit. This allows the telescope to see changes in radiation as different parts of the planet come into view, corresponding to a longitudinal map of planet temperature. Such changes are known as phase variations, referring to the star illuminating the planet at different angles just like phases of the moon.
An example of what can be drawn from this is found in the intriguing case of a planet known as 55 Cancri e. 55 Cancri e is a planet roughly twice the size of the Earth on an orbit so short that the scorching hot world circles its star in just 17 hours. This close proximity to the star makes 55 Cancri e tidally locked; like the moon orbiting the Earth, one side of 55 Cancri e always faces the star, giving a hemisphere of perpetual day and one of never ending night.
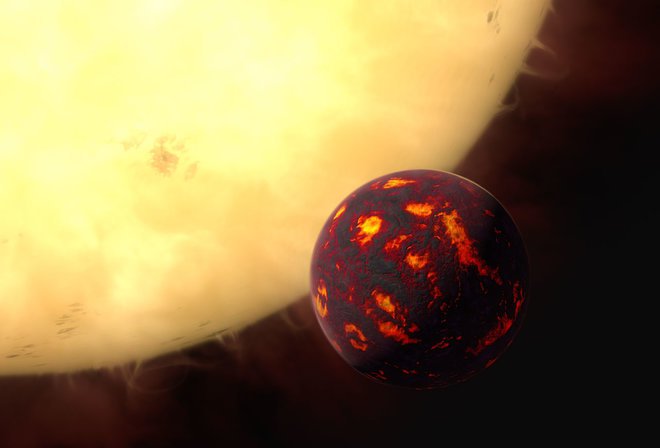
Super-Earth 55 Cancri e orbits in front of its parent star in this artist’s illustration.Image credit: ESA/Hubble, M. Kornmesser.
It would be logical to assume that the hottest spot on 55 Cancri e would be the center of the day side, known as the sub-stellar point. This would be visible just before the planet ducked behind the star.
However, a 2016 paper in the journal Nature, led by Brice-Olivier Demory from the University of Cambridge used NASA’s Spitzer Space Telescope to map the phase variations of 55 Cancri e. To their surprise, the hot spot was shifted by 41 degrees east of the expected location. This suggested either 55 Cancri e had an atmosphere capable of redistributing the star’s heat or perhaps the baking surface was molten rock that pushed the hottest material along a river of lava.
The result from 55 Cancri e shows that ARIEL will not be the first attempt to study exoplanet atmospheres. However, it will be the first mission entirely dedicated to this task. This comes with serious advantages.
The first forages into exoplanet atmospheric data came from the Hubble and Spitzer Space Telescopes and ground-based observatories. These have been enticing but sparse, with data from only a handful of planets smaller than Neptune.
The upcoming (but, alas, just delayed until 2020) NASA’s James Webb Space Telescope (JWST) and the European Extremely Large Telescope (E-ELT) will also be powerful observatories for exploring exoplanet atmospheres, but these are general purpose facilities whose high demand will give time for just a few tens of possible planetary targets.
To truly explore the composition of exoplanets, we need an order of magnitude more examples of atmospheric data. Without this, we risk building our models of planet formation on a handful of observed compositions that may not be typical, or only occur in particular situations. ARIEL aims to observe around 1000 exoplanet atmospheres, probing the gases enveloping planets from Jupiter to super-Earth in size.
“With the current data sets, we have unsettled questions including why some planets have flatter spectrum (less evidence of light being absorbed by different molecules) and others with distinct absorption features, and the diversity of atmospheric inventories and profiles is not well understood,” explains Yuka Fujii, Associate Professor at the Earth Life Science Institute (ELSI) at the Tokyo Institute of Technology. “The large number of planets Ariel will give us more clues.”
As a mission with a dedicated task, ARIEL is also fine tuned for the job.
The telescope can simultaneously examine a broad range of wavelengths covering all the major expected atmospheric gases such as water, carbon dioxide, methane, ammonia and hydrogen sulfide and cyanide through to the metallic compounds.
Simultaneous measurements are particularly exciting, as stars are rambunctious plasma balls and stellar activity in-between observations may interfere with being able to directly compare different wavelength observations.
While the atmosphere is potentially a good place to hunt out biosignatures, directly spotting biological action is not a target for ARIEL. The planets ARIEL will be studying are hot worlds which orbit close enough to their star to have equilibrium temperatures (the temperature at the top of the atmosphere) above 660°F (350°C).
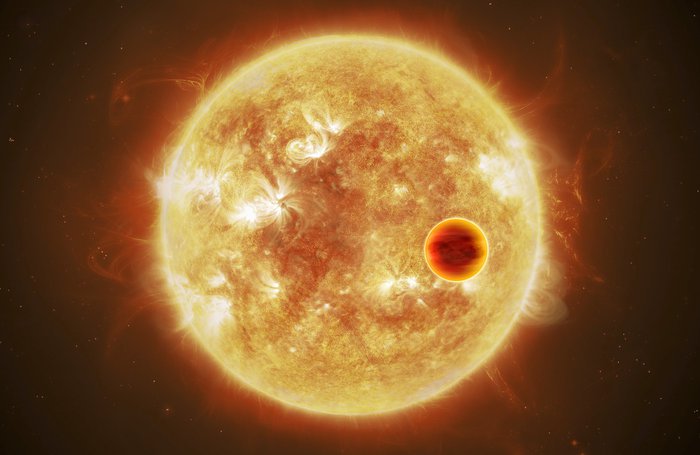
ARIEL will focus on hot planets that orbit close to their star.Image credit: Artist impression, ESA/ATG medialab.
The advantage of the high temperature is that the atmosphere should reflect the composition of the planet, whereas a cooler world might have many of its molecules in solid form or in condensed clouds, hidden from ARIEL.
Speaking to the journal Nature, the principal investor for the ARIEL mission, Giovanna Tinetti said that “ARIEL can really give us a full picture of what exoplanets are made of, how they form and how they evolve.”
Probing the main composition for the planet makes the data from ARIEL invaluable to understanding how and where planets formed. This last point is particularly intriguing, since results from our planet hunting telescopes strongly suggest planets do not stay where they are born.
The first discoveries of Jupiter-sized worlds on orbits much shorter than Mercury was highly surprising, since there should not be enough dusty building material so close to the star to create large planets. This advanced the idea of planetary migration, where planets form on one orbit and then move to another.
What is not clear is where these planets formed and when they began their migration. Unpicking the planet composition will help pin down their trajectories, since different elements condense into planet-building material at different distances from the star. A planet rich in water vapor, for example, likely formed far from the star where it was cold enough for ice to freeze.
Mapping the evolution of planetary systems has relevance for more temperate worlds, as the composition of planets on Earth-like orbits will dictate whether they can support processes we use for life, such as a carbon-silicate cycle and magnetic field. As the first dedicated atmospheric explorer, ARIEL will also act as a pathfinder for future missions that may one day be able to sniff the gases around a habitable world.
Whether your interests lie in composition, planet evolution or habitability, ARIEL will be the one to watch.
A wonderful overview of the different exoplanet missions, including ARIEL, Plato and TESS, can be found on David Kipping’s “Cool Worlds” YouTube series.