

We welcome to SAGANet Dr. George Cooper, Senior Scientist at NASA Ames Research Center, and an expert in meteorites and their potential role in the emergence of life on Earth!
You can read more about Dr. Cooper and his work
here.
Greetings, friends of astrobiology. Welcome back to a new episode of Ask an Astrobiologist. This program is the show of the website SAGANet.org, where we celebrate science, diversity in science, and scientists. This program is made possible by contributions from the NASA Astrobiology Program, ELSI, the Earth-Life Science Institute, and the nonprofit Blue Marble Space. My name is Sanjoy Som, but before we start, this is your background quiz, like every month. Mike, if you could put up the background from last month. It is, actually nobody got it right, there was no suggestions for what it is.
Other Recent Episodes
How & When Do Molecules Become Life with Dr. Henderson "Jim" Cleaves
From Deep-Sea Treasure Diving to Exploring the Surface of Mars with Dr. Michael Meyer
Exobiology to Astrobiology: the Evolution of a Scientific Field with Dr. Linda Billings
I was asking you guys to kind of identify what the little mounds were on the bottom left of the image. Those are actually modern stromatolites. Those are microbial mats that grow up towards the sunlight, of course, and they form mounds. They're modern analogs of some of the most ancient fossils of life we know on Earth. The ones from the background from last month are in a biological reserve in Mexico, called Cuatro Cienegas, if I pronounce it right. Some of the oldest ones we have found in the fossil record are over 3.5 billion, and that's with a "b," years old. So really really ancient forms of life that are found today in analog environments.
Speaking of analog environments, what is my background this month? You can see this cold ice desert. It's a location where scientists have gone to test instruments that are destined for Mars exploration. If you know the answer, put it on #AskAstrobio on Twitter or on the SAGANet.org chat, and we'll give you a shout out next month if you get it right. Speaking of very old stuff, this month we're going to talk about even older material, meteorites, and for that we are very lucky to welcome to the program Dr. George Cooper, who is a senior scientist in the exobiology branch at NASA Ames Research Center in California. Welcome, George. How are you?
Oh, fine, Sanjoy, thanks.
Like we like to do in this show, we first rewind the wheels of time a little bit. We are curious about how did young George emerge to become the scientist he is today.
Well, I hadn't really discovered scientists, science until basically college, and just sort of fell in love with chemistry instead of other subjects in undergraduate. From there, one thing led to another.
Why choose meteorites? Chemistry is a very, very broad discipline, right? What got you interested in meteorites and their potential clues to the origin of life?
Well, in graduate school at Arizona State University, my professor, well, my eventual professor, major professor was John Cronin, who maybe some people in the audience have heard of. The reason I ended up with him is that I'd always liked astronomy, having a telescope and things like that. Like I said, in undergraduate I liked chemistry, so it was trying to find an area of study in graduate school that sort of melded those two things together.
He was the perfect person, because meteorites, undoubtedly you cannot talk meteorites without talking astronomy and the solar system. At the same time, the work is basically analytical chemistry. You know, I would say John Cronin, and then there's the Arizona State University meteorite center, I think the world's largest university meteorite center. It sort of came together from there.
That's very exciting. It's clear that you're an astrobiologist. Interests from astronomy and chemistry are merging to guide your field of research today. Let's talk first about meteorites. Could you describe to us what they are, how different they are? You know, there are iron meteorites, there are chondritic meteorites. What are the two differences and where do they form? Why are those important to study in the first place?
Well, the astronomers would take you back to the beginning of the solar system, and now they have photos, actual photos of other forming planetary systems that form out of rock, dust, gas and coalesce into larger bodies, planets, comets, asteroids. The meteorites that I study, when most people say the word meteorite, they're usually thinking of asteroidal meteorites. Asteroids, like comets, are leftover bits of planetoids that didn't form the larger planets like Earth.
Comets, you could have meteorites from comets also, but I think the historical problem is, if you picked one up, you wouldn't know whether it was a comet meteorite or an asteroid meteorite. The meteorites we do have appear to match the spectra of asteroids. Right now, carbonaceous meteorites in particular are pretty much almost all that scientists know of. Probably came from the asteroid belt, from the initial formation of the solar system.
The organic chemistry that's happening in meteorites is not something that happens once the meteorites have arrived on Earth, right? There's organic chemistry that can happen if the meteorites have ice when they are in the faraway solar system?
Right, and even before the bodies of the asteroid, the parent bodies of the meteorite formed, people for example here, but over the years, in Leiden, have shown that on the interstellar grains, the grains that eventually coalesce to become asteroids and comets, even there you have chemistry taking place. You have carbon, oxygen, nitrogen, and a source of energy, photons, radiation. Organics are even made before then. There's a big group here at Ames, Scott Sandford, Lou Allamandola, Michel Nuevo, who do this all the time. They form organics simulating the conditions of interstellar grains, 50 degrees above absolute zero, things like that.
That's probably I would say where the initial organics are formed, eventually to be in the rocks that we have in the lab. After that, the grains, as I say, they coalesce, and there's further chemistry once that water warms up more. If you have chemicals in water, you're going to get more chemistry. So for example, glycine, the smallest amino acid, easily form from formaldehyde, cyanide, and a few other, a couple other things. They're all seen in interstellar space. All the chemicals that amino acids and therefore us, we're made of, are out there.
Once they land on, freeze out on these grains, formaldehyde, cyanide, aldehydes, and ketone, once they form on the grains, as I said before, there's chemistry. When they warm up, incorporate into the larger asteroids as evidence for aqueous alteration, there are two. So two I would say primary sites for organic formation.
We are really a LEGO structure of organic chemistry. If some of those fundamental organic compounds came from meteorites, which are not formed on Earth, but formed in the early solar system, that really means that we are star dust, which is quite humbling, I think, right?
No, that's ...
No, go ahead, sorry.
Yeah, that's the same chemicals astronomers see wherever they look in the universe, which is a good sign for potential life in other places.
Life could have been, if life exists on Mars and elsewhere, of course you have no confirmation of that, but those early, those planets were also subjected to the same early bombardment as Earth, and therefore they also were contributed organic material. In your view it's possible, given the right circumstances, and it's a big "if," that life could have emerged on other bodies, other than Earth, of course.
Oh, yeah, of course. Even Mars last year, I think 2015, maybe it was, the finding of organics on the surface by the Rovers. The paper, I think it was the Journal of Geophysical Research, a big group, said that, well, the organics that we're finding are probably either meteoritic or from some indigenous Mars process. As I say, how the astronomers see these compounds, every place they look, and now obviously land on Earth every day, or we wouldn't have them, the rocks in our labs. But now on Mars, years ago, we saw a comet crashing into Jupiter, so yes, the potential, in addition to the meteorite organics, the further chemistry on Earth, or maybe some also indigenous Earth compounds. Yeah, I think there's a great potential for other life.
All life, potential life in the solar system is shopping for organic matter at the same grocery store, right?
Mm-hmm.
How does one find meteorites on Earth, and how do you differentiate them from other rocks?
Well, there are big groups, for example, that go to the Arctic, and in ice-covered, snow-covered backgrounds, like the one behind you.
Exactly.
I've seen some of the pictures, and they're pretty easy to spot. Most meteorites are darkish and against the snow. You can readily pick them out, but also they're seen to fall a lot of places, and if you're careful and you follow the trajectory, you can find them yourself. For example, the Murchison meteorite, the most famous, was found in Murchison, Australia, in 1969. People saw it fall and saw a big debris field. That's the major type of discoveries. Antarctic, a lucky find. They're transported to museums, most of them, the ones that aren't privately found. Scientists, me and others, can request from museums or Antarctic meteorites again, from, say, the Johnson Space Center, which has a huge collection. Those are the major ways to get them for scientific research.
You have actively worked on the Murchison meteorite itself, which is really cool. Could you tell us a little bit about how you feel when you receive a sample that's as famous as the Murchison, perhaps you can describe to us also why it's famous and what goes through your mind when you hold this rock that 4.6 billion years old?
Well, we had an outreach the other day with students from one of the high schools, somewhat sort of local high schools, and I had powder that is no longer used for research, meteorite powder that we grind up and extract out the organics. But what I did was let them smell it, because if you shake it, the particles are still breaking down to this day, and the original gases from the solar system come out. Most students say it sort of smells like dirt, maybe wet dirt in the country when you walk along the road.
The feeling you mentioned is still something else to think that you're actually looking at, smelling things that are actually closer to 4.6 billion years old. Then it's a process of extracting out organics with normal analytical chemistry means. As far as Murchison, the reason it's I would say more famous is because when it fell in '69, there had been meteorites of course a long time before that, and there had been analysis before that, but mostly they were very contaminated.
Of course, the earlier people had found some indigenous compounds, but a lot were just earthly contaminants. Murchison, as I said a few minutes ago, it was actually seen to fall and picked up very quickly, and on top of that, it had many, many organic compounds. It sort of stood out right away, and the analysis began and people discovered amino acids in it. The older meteorites were thought also to have amino acids, but again, that contamination problem. Murchison sort of settled that question once and for all, and that's why it's the more famous.
You bring up a good point, in the sense that Earth is covered with biology, right? If a rock from space falls on the planet, then the biology that's near it could contaminate it. How are we sure that these organic matter that we find in meteorites are not just Earth contaminating the rock?
Right, right, in almost every meteorite I've looked at, there's Earth contamination, at least some. There's one that's very clean, but most, right, life loves any carbon source. The way people have discriminated against indigenous versus contamination, one of the principal methods was isotopes, and people watching probably have loosely heard of isotopes, different weights of the same compound. For example, carbon, most carbon in nature is 12, weighs 12 on the periodic table. There's a little bit of carbon 13, I think, around 1 percent, I forget the exact number.
With mass spectrometers, very sensitive mass spectrometers, it's quite easy to tell if let's say the carbon in glycine has the Earth ratio of carbon 13 to carbon 12, or if it's higher relatively in carbon 13 than to 12. That tells you right away, that is not from Earth. Geochemists are very good, even with only earthly samples, they can take isotope measurements, let's say of a rock in California or a rock in India, and in some cases tell you what country it's from or what region, rather, it's from, simply by the isotopes and how it was made.
The astronomers see that in space, many compounds are fractionated, that is, these organics I mentioned a while ago, many are higher in the heavier isotopes, of carbon, hydrogen, nitrogen. When they're incorporated again into the parent bodies of the asteroids, fall to Earth as meteorites, that signature, a good deal of it, is still there. That's number one. The second best way is in some cases just as good, is to look for organics that aren't used in biology. That's what people have done a lot since Murchison, look for strange amino acids, because asteroids wasn't a biological process.
It would make glycine with two carbons, all the way up to ... The space process is abiotic, non-biological process, would make from carbon number 2 to carbon 15 for amino acids. It's just thermal dynamics, whereas life on Earth uses very specific amino acids, very specific weights. Life, your body, bacteria doesn't want to make every possible isomer of a compound up to C-10. There's simply, that's a waste of energy. Again, the nonbiological, abiotic process in asteroids, probably comets, make all, including weird ones not used. That's how you tell if it's really from space, is nonbiological compounds.
That's so cool. Meteorites are really the history book of the solar system, and you and your colleagues learn the language of the rocks, really extract the story of what that means for the formation of planets, but also for the origin of life. It's just a fascinating field. Many years ago, I was lucky enough to be in Washington, D.C., and was visiting the meteorite collection you were talking about, and I held in my hand ALH 84001, which is like the meteorite that we know came from Mars. There's not only meteorites that come from far beyond in terms of asteroids that feed the Earth, but also the different planets are exchanging rocks with each other. Have you worked with Martian or even lunar samples?
No, not with the ALH you mentioned, nor moon rocks. My research is primarily the asteroidal meteorites.
Okay, and the fact that rocks can transfer between planets is also I found mesmerizing, because you can tell some stories about the Martian atmosphere from the atmosphere that's trapped in bubbles that form on Mars and that rock was transported to Earth, from which you can analyze using the tools you mentioned. The analytical techniques and the precision you need to make such conclusions is incredible. I was also quite amazed to hear about your colleagues that simulate the solar radiation inside the laboratory to create the conditions that leads to the development of organic matter, that then you can take in the laboratory using your techniques to see what's there. Then does that conform with what we can expect? Wow, it's really cool stuff.
When you were a student, I'm sure like all of us that's been through college, it's not an easy path to navigate, especially when you're after a PhD. I was wondering if you benefited from mentors in that process. Any people that contributed, that helped you out to become the scientist that you are today?
Well, again, graduate school, your best mentor is going to be your professor. Undergraduate, you do have of course professors that some you're closer to than others. Of course, there's always help along the way, but for getting settled in your particular field, for example, the organic analysis of meteorites we're talking about now, the major professor of graduate school is I would say the chief mentor and so I was lucky. I had what I call the professor's professor in John Cronin, who took his work very seriously, and he sat there all day and get everything "i" dotted, everything would be correct when he got up.
I would say that mentor-wise, but you know, at ASU, as far as being in meteorites, you know, you had his postdoc there, Sandra Pizzarello. You had the ASU Meteorite Center, with Carleton Moore and other people there, who this was serious business for them. That always affects a student, as you probably know. It's the whole community of meteorite, in my case the organic analysis of meteorite group.
What did you do after you finished your PhD with an expertise in meteorite studies?
Well, I came to Ames as a postdoc for Sherwood Chang, who's one of the founders of astrobiology. He really worked for years to focus research, get funding for research, and he was the branch chief at that time of the exobiology branch here. He retired years ago. I was his postdoc, so a few years postdoc, and then a position came open, I was lucky to get the research scientist position here. That was pretty much just the trail from graduate school.
Cool, cool, cool. Let's see. The academic environment is one that is changing, and I was wondering if you have any recommendations for minorities who are interested in science and want to become scientists and get PhDs in science.
Well, I guess if you start at undergraduate, the advice, a lot of the advice is the same for everyone. Find opportunities, ask professors, counselors, about internships of things you might be interested in. Let's see. Now there's the Internet, you know, to search. Minority, for example, I knew people at Howard University and Texas Southern. It's always good to try to reach out to people like that who can tell you how they operated around the system, you know, what made life a lot easier. That and I always half-joke, that and get lots of sleep, but health. I would say if you have an idea of what you want, then there's nothing like getting internships, finding the information. Most professors at their schools should have an idea of internships and opportunities. I think that's huge.
Yeah, and students have really nothing to lose for reaching out and contacting an expert. "Hey, I'm really interested in this. What can I do to advance my career?" Most of the time, you'll get a response. All of you who are students who are watching this, don't feel shy about connecting with a scientist.
Exactly.
Most likely they'll be delighted to reply. What do you find most exciting about being an astrobiologist?
Well, again, it goes back to what you mentioned, is looking at these 4.57 billion year old rocks. That is still to me amazing. That is number one, the fact that you can look at something that formed before anyone thought about humans, before there was any thought about, before the Earth actually formed. Now again with the space telescopes, it's just not some delusion. You actually see the same process happening all over the place, over and over again. It really drives home the point that you are looking at formations of planets eventually to be people and bacteria and crocodile. You know, it's just amazing. That I would say is still number one.
Can you paint in your mind and share that with us, perhaps, how you think life arose on our planet? What kind of environment?
Yeah, boy, that's a Nobel Prize question.
Right, that's a tough one.
Maybe in three years, I'll give you an answer. But that's the golden question, you know. We see the compounds, organic compounds in meteorites, but it's obvious that it took from there some more, some additional chemistry on the Earth. For example, right now, we don't see long polymers of proteins, you know, made of amino acids, which is in everything that's life. There's a very small two amino acid peptide, very small piece of a protein, but that's in very trace amounts. It had to take more chemistry from the Earth, and probably additional chemistry in hot regions. People are doing hydrothermal vent simulations, people like George Cody at Carnegie.
Years ago, researchers took amino acids and simply put them on what they simulated to be hot islands, ancient volcanic islands on the ancient Earth, using just heat. You can form linkages, you can join compounds together, which is necessary before you get life. You have to join individual small compounds together, and they could do that with simply heat, by mimicking the many, what had to be the many volcanic hot areas on the ancient Earth. Heat is just the simplest thing to do. I don't know of a complicated scenario for life. People are working on it, but I would start with something like that. We'll, as time go on, ideas will get better and better.
Yeah, it's an active area of research. If I understand you well, the seeds you need to get life started on a planet requires not only solar system chemistry, where you have cosmic radiation that's interacting with ice in the meteorites to create this organic matter, but also the geochemistry, the chemistry that's happening at the interaction of a hot terrestrial or planetary surface, interacting with rocks and water and the chemistry that's happening then, putting those two together, and with the proper conditions, could then arise biology, right? So it's not only a closed planetary ecosystem that leads to life, it's this interaction of the planet with its outer solar system environment.
Exactly. Even though I'm fascinated with meteorite organics, this big Earth wasn't sitting here for nothing billions of years ago. Like I said, undoubtedly there was further chemistry, maybe different chemistry. The organics in meteorites, you can make very fragile compounds that you needed, probably needed for life, for example, pyruvic acid, oxalic acid, things like that, because you're forming them out there, as I said, at 50 Kelvin. Very cold. There are radical compounds that are very stable in interstellar space. When they fall to Earth, they're very reactive. Right away on the Earth's surface, they could have done more chemistry, but again also linking monomers, linking amino acids together, sugar or sugar-derivatives together, to make our larger, the larger polymers we're made of.
Right now, it looks like that had to be an Earth process. Whatever the mechanism, like I said, I sort of favor warm, maybe even hot, at least localized. The ancient Earth, for organics, couldn't have obviously been terribly hot or you wouldn't have had organics, but localized at just the right temperatures. There must have been a very, a huge amount of different temperature regimes, for linking compounds together, I think that would be, the right compounds, and there are many in the meteorites and who knows what were made on Earth. There are the right compounds that if you did have a warm or hot environment, that easily could link, compounds could have been linked together to form larger polymers.
Fascinating. Could you take us to your lab and describe to us what you do in the lab? What kind of instruments do you use to make such detailed measurements?
A few different types of instruments and techniques. The first is really boring analytical chemistry stuff.
Oh, don't say that.
You have to prepare your sample, you have to extract with a solvent, it could be water, it could be a more nonpolar solvent. First you have to extract the compounds out. There are a lot of salts in meteorites. So that's, to keep the story short, you couldn't do the type of analysis I do in the mix of all the salts. For example, in Murchison, and a lot of carbonaceous meteorites, there's lots of sulfate, SO(42-). Murchison, a lot of it is magnesium, calcium.
You have to what we call de-salt. You have to remove those to the side, to get at the organics. Here's where the boring analytical preparation techniques come in. You use resins to de-salt, and you go on and on and it can take forever for one sample. The instrument that finally tells you what the organic is in my case is mostly gas chromatography-mass spectrometry, GCMS. A little bit of liquid chromatography, but that is my, has been my major analytical instrument that actually identifies compounds. I use others for other reasons.
Can you, could you describe what a GCMS is and does in just a few minutes?
Okay, a gas chromatograph, the GC part, you inject, after you've done all the preparation, you inject the sample. The word gas implies, of course, a gas phase. It vaporizes your sample, puts it onto a GC column. A column is a long, glass capillary tube, coated inside with an organic phase. Your compounds, after you inject, land in that long column, and these columns can be 25 meters, 50 meters. Often I've used even longer GC columns. They land in that phase.
Again, back to the gas part, you start heating your gas chromatograph, so your columns inside the gas chromatograph. The organics are now, they've landed on that column. Now you start heating that column, and eventually things boil off. They boil off at different temperatures, because most of the audience knows about a boiling point. Water boiling point is 100 degrees Celsius. The organics on this column, as you slowly heat up your column, they come off at their own particular boiling point in that particular organic phase of the column.
That's the major way to separate organics. They boil off, they're swept out of the column. You're always running a gas through it, an inert nonreactive gas that doesn't react with your compounds, for example, helium or hydrogen. Because each compound has a different boiling point, you could imagine, when these compounds are leaving the column, going to the detector, the mass spectrometer, you'll see each compound come off at a different time. In other words, this gas chromatograph is taking this big goop of a mess of organics in the meteorites, and separating it so you can identify one by one the compounds. You couldn't do it if you simply had all the organics just sitting there in one big mixture.
After they go to the mass spectrometer, the mass spectrometer can tell you the molecular weight of the organics, and it also fragments the compounds. It shoots a beam of electrons at each compound, they break apart, fragment, but they do it in different ways. That's called a fragmentation pattern. The fragments are an ID, fingerprint, of each organic. That's two ways to identify. The retention time, or the time it takes each compound to boil up and come out, and its fragmentation pattern, how it breaks apart. You know that because you run several standards either before or after you've analyzed your sample, and if that standard matches, then you can go back to your sample and say, "Aha, this one had the same retention time and the same fragmentation pattern."
GCMS, the gas chromatography mass spec, is what was used for I think the first big Olympic cheating scandal. I think that was 1990s, maybe Sanjoy, well, you probably weren't born then. I think it was the '90 or '92 Olympics, there was a big doping scandal. And a gas chromatograph mass spec did exactly what I just described. It identified the compounds and so if you were caught, you couldn't say, "No, no, no, the GCMS was wrong," because it's really tough to be wrong when you have a standard and all the properties are identical. It's pretty useful. For example, the water in the background of Sanjoy there, when you melt it, you could analyze it by GCMS to tell what compounds are there. It's a pretty useful technique.
That's so cool. I could talk to you forever about meteorites and organic matter, but I think it's time that we opened the floor up to questions. I'm going to turn to my question screen, which is right here. The first question is from Michael Wong: "What is responsible for choosing the chiral 'handedness' of organic molecules in life?"
That's another golden question. No one knows yet. Scientists know why it works so well. What he's talking about is if, I don't know how diverse the audience is, but if you put up your two hands, you could imagine, pretend, that's an organic compound. You have a left mirror image and a right mirror image. Well, for example, in RNA and DNA, only one of those mirror images of the compound, in this case, let's say ribose in RNA, only one is used in those long strands of RNA and DNA.
Most people from school, high school, have seen the long strands, you have an RNA, a base and phosphate, and it repeats. RNA, ribose, phosphate, base, and it goes on and on and on. But only one of those ribose, only the D mirror image is used. That's what caller Mr. Wong is asking about, how in the world can you get just one? Because if you go in the lab to make, let's say ribose, nonbiologically, like what happened before life, it wasn't a biased process. If you go in the lab now, and do the same thing, you'll get both. You'll get two hands, just like flipping a coin a thousand times. You can't get one coin, only heads.
That is a good question. How did one get picked out? From what we're seeing now with compounds close to sugars, and what my old professor and Sandra Pizzarello saw back years ago, a paper published in Science in 1997, was that some of the amino acids have a slight bias of one of the mirror images. Sometimes the amount, the excess of one of those mirror images vary. It's the L amino acid, which just by coincidence is the one your protein is made of.
Lately we see sugar derivatives, cousins of ribose, that has a lot more of one mirror image, and that's the D mirror image, and maybe it's coincidental now, but the D of the sugars is what life uses mostly. Is that a hint to the answer to that question? What else happened on the ancient Earth? That, what I described came from space, comes from space, what else happened on Earth? People are looking at that. Maybe if this worked, we could do more of it, and see the same thing over and over. I saw that phenomenon since 2001, but we just published it, but I've seen the same phenomenon in these sugar derivatives for over 15 years. People wondering, why the heck aren't you publishing it? You still, I still, you should still look at it in different ways.
The reason it works, I say at first, scientists weren't sure about how it happened, but they know that you can only form, let's say, RNA and DNA if you have one mirror image. That's why it works well. You can't have both, what's called a racemic mixture, you can't have left hand and right hand ribose and go and try to make your DNA. Jerry Joyce at Scripps showed years ago, I think it was '84, a paper in '84, that you don't get very far if you mix both of those mirror images. We know why it works well, but the initial start is still a question.
Cool. Thanks for that, George. Our next question comes from Janet Ashley Pollock. Hello, Janet, thank you for your question. She asks whether comets could also deliver organic material on Earth.
Yes, we just, you know, don't have what we can actually say is a comet, a bonafide comet. Like I say, some asteroids were probably comets. Who knows what, organically wise, what the difference is. We just can't say. There was a paper last year or a few years ago, last year, I think, where they identified glycine on the famous comet now 67P, where the European space craft is landed on. Glycine is the smallest amino acid. They identified small organic molecules, CN, cyanide, a few others, but the larger more, what we think of as biologically relevant, I don't think is known about comets yet.
Even if there were only those small molecules, formaldehyde is one of them. There's formaldehyde is just CH20. HCN, cyanide, another compound. Even if this only, they are the only ones on comets, if they landed on ancient Earth, the chemistry you could get, the further chemistry, the building of larger compounds from that, I think would be pretty significant. Again, if you have formaldehyde, CN, which are in comets, right away, not right away, but pretty much, you're going to get glycine and other amino acids. Just the small molecules would have been I think huge, as far as further chemistry from comets.
Thank you. The next question is from Gina Riggio. Hi, Gina, thanks for your question. She asks, "What is an example of an unexpected organic compound that you have recovered from a meteorite?"
Oh, the most unexpected. I would say it was an organic phosphorous compound that was fairly unusual bond. For example, DNA and RNA are organic phosphorous, but that's not, as far as we know, not in meteorites. That's phosphorous with four oxygen around it. The RNA, each phosphate is bonded to a ribose, and then another phosphate and then another ribose and on and on and on. That's PO4, phosphorous with four oxygen. The most surprising that I can remember is, the organic phosphorous we saw years ago bonded directly to a carbon, and it has different organic groups attached to the carbon. They're called phosphonates, not phosphate, but phosphonates. I think that, compound-wise, not phenomenon-wise, but compound-wise, is probably the more surprising.
Cool. Janet asked a question again, about whether you have any, she's Canadian, and she's asking if you have any Canadian colleagues who do some of the early life, early Earth type studies.
Not myself, I do not, but there are people in Canada who like meteorites also and analyze organics. But not personally, no.
Janet, you should check out the Center for Planetary Science and Exploration, which is in London, London, Ontario, I think? They might have, it's at Western, they might have a department there that you might find some collaborators. The next question is by Abhishek Soni. He asks, "What can an environmentalist do with astrobiology?" If you're a modern ecologist, environmentalist, how can that give you insights into astrobiology? I think that's where that question is going.
Well, I haven't thought of the environmental connection before. As far as astrobiology, you said? Between the environment and astrobiology?
Yeah.
Can you, yeah. No, I can't think of a, when I think of environmentalism, I'm thinking of trying to save the planet from its current, you know, head towards destruction, climate change and the rest. I'm sure there must be some connection. The astrobiologist that I spoke of before, they go all around the Earth searching for meteorites. Obviously the ice melting where they go collect meteorites, so I'm sure they would have a really strong bent on that particular question.
It's true, and analog studies, Abhishek, are a modern way of studying astrobiology. Look at analog sites that are similar to some conditions you're exploring in other worlds, and if you have some expertise in the ecology or the local biogeology that's going in there, then you can make some educated conclusions about the plausibility of that environment to be similar to one elsewhere.
There are so many questions that are pouring in right now. Thank you all for all these great questions. We're probably not going to have time to get through all of them, but we'll do the best we can. What is the next question here? Oh, it's a question by Jacob Haqq-Misra. Hello, Jacob, thank you for your questions. He asks, "What are the main geochemical differences between meteorites that originate from Earth compared to from Mars?"
You mean the meteorites that fall to Earth?
Yes.
Martian meteorites that I know of, again, I haven't studied, but I think the majority are more rock-like. One or two have, they have found traces of organic compounds, but they look like they come from a heating process, an igneous process. Certainly the big complex mixture or organics that we see in asteroidal meteorites that fall to Earth, cannot be seen in Martian meteorites. I would say they're more rock-like, more planetary-like, less organics.
Yes, meteorites from Mars are essentially like lava flows that then cooled off and became rock that were then ejected by some sort of impact and landed on Earth. Whereas meteorites are more primitive silicon material that are what the planets are made of in the first place. I think that's what you're after, Jacob.
Yeah, more pristine.
More pristine, that's it. The next question is by Graham Lau. Hello, Graham, thank you for your question. "What do you think the odds are that we have Venusian meteorites already in our collections, but just don't know about them?"
Oh, well, the ones in the collections, they're pretty well characterized. Every now and then I will hear something about a meteorite, possible rock from Venus, but I don't know the people who think, the geochemists, the geologists actually, who think about that type of thing, I don't know what the latest count is, but I do hear about that now and then. I just couldn't give a good answer.
It's a good question, Graham. I don't know either. Venus meteorites, that would be cool.
There's probably some rocks from a lot of different places on Earth, we just wouldn't recognize it, if we stepped on it.
Yeah, because you have to find them in specific conditions to recognize them as meteorites, it's not always obvious. The next question is Gina again, she ask a very good one, in terms of, you were talking about the column in your lab that's like 25, 50 feet, or meters, or even longer. So she asks, "How does that fit in your lab in the first place? How many compounds can you separate using that technique?"
Well, I plan to ask the Center management for a nice long lab. No, the columns we're talking, the ID is a millimeter less, the internal diameter, so they're tightly coiled. They're made of flexible polyimide material. You can imagine, take 50 meters and coil it up into a circle of about, let's say a foot across. That fits in your gas chromatograph oven.
Gotcha. How many compounds can you separate? Like a hundred in the sample?
Oh, more than hundreds, more than hundreds. Yeah, in a single run, you could have, you know, you can't even count the number in the meteorite, it's just a strange number.
Sukanya Singh asks if you've ever detected a compound that is not organic and that you're like, whoa, that's a really weird one. That should not belong here. Or is everything that you've discovered kind of expected from the cosmic chemistry?
I do see nonorganic compounds now and then, as just simply you don't get all of the inorganic compounds out. For example, sulfate, SO(42-) I mentioned. You try to get out most, as I said, but you see sulfate, phosphate, traces of it, sometimes in your analysis. Sulfite, SO3, and there's probably one or two others. Those are the principal nonorganics that I'll see now and then.
Cool. Kai Pisarek asks about the enantiomeric excess, so the excess of one chiral molecule versus another. What is the excess, I guess in meteorites, and couldn't you use that excess to say something about biology? How big does the excess need to be in order to make conclusions about biology?
Okay, well, going back again to before I started, and I mentioned the '97 paper, Cronin and Pizzarello. Before that, there was a paper looking at biological amino acids. Ingall and Mackell, and I forget the year, around 1990, of alanine, a biological amino acid. Again, people are always leery of biological, if you say there's an enantiomeric excess, but it was good work. We'll have to see about more about the biological amino acids. When you get to the '97 paper, Cronin and Pizzarello, as I said, some of the strange amino acids that are not in biology, that's there's no question that they came from space, so if you see an enantiomeric excess, you can say that's indigenous for a fact.
Those enantiomeric excesses, or in other words, one hand more than the other, the excess of one mirror image of the amino acids, back then they were in the range anywhere from 1 percent, I think, up to 10 percent. This was more than one amino acid. They concentrated again on the nonbiological and nonprotein amino acids. That's another thing. You want ones that aren't laying around everywhere in proteins. They were in the range of 1 to 10 percent. Other people have done, looked at those compounds, too. The paper, boy, it's early morning, I forget the year of the paper, but Glavin and Dworkin, I think Jamie Elsila, too, they did a big survey of meteorites and they found large excesses in some of the same amino acids. I think they went up to 20 percent. So 20 percent excess of one enantiomeric, one mirror image.
Then Pizzarello, Sandra Pizzarello found 50 or 60 percent excess, you know, one over the other, in other larger amino acids. I think these were isoleucine derivatives. Mostly with the amino acids, though, you're talking about that range of 5 to 10 percent excess, just generally. The ones we saw last, now switch to sugar derivatives, away from amino acids, so last year in June, we published that. We went compound by compound, increasing carbon number, so we went from glyceric acid, so instead of the sugar, glyceraldehyde, if you have your organic chemistry book, the oxidized form is glyceric acid. You add an oxygen, and you get an acid from that sugar. We started with glyceric acid, which is three carbons.
Then we went to four carbons, to five carbons. Five carbons is where you get to the relative of ribose, the very close structural analog of ribose. Then we went to six carbons, where instead of glucose, you have gluconic acid. Most of those six carbons are rare on Earth, gluconic is not. Back to the question. At the first carbon number, there were glyceric, it was fifty-fifty. No matter what meteorite you looked at, fifty-fifty. Now if you got a very contaminated meteorite, you knew it was contaminated, you can see a lot more D-glyceric. But for normal, mildly clean or super-clean meteorites, the three carbon was fifty-fifty.
As we went up in carbon number, that spread increased. We went to C-4, it was three to one D [inaudible] and threonic. We went to the five carbon, the ribonic acid, the cousin of ribose, that was eight or nine to one D. You went up to the relatives of glucose, and it was only D. We didn't see any of the other mirror image, the L. I would still not believe it, except the weird non-earthly ones are the same way.
There's no strict boundary, right? There's no strict definition that above 50, it's biological, and below that it's not. It really depends on what compounds you're talking about and so on, right?
Oh, right, right. We're talking about on a molecular basis, what's found in life and therefore can contaminate your experiment, and what's not found in life. They all show this increase in excess of one mirror image. I should modify one ...
We're running close on time.
Oh, okay, okay.
I just want to give the opportunity of having one last question before we end. That is, Damian Sternmann, hi Damian, thanks for your question. He is asking perhaps a more speculative question, because life on Earth and life on other terrestrial planets probably came from the same building blocks. He asks, "Do you think, and speculatively, would alien organisms also use peptides and DNA and similar stuff that we're made of?"
That's another good question, but I've heard astronomers say, we see the same stuff everywhere. They look in space. I think there's a very good chance, yes. They might use a different ...
Figure this one out, Damian, and then let us know. That's Nobel Prize type results.
Yeah, they might use a different mirror image, but same compounds.
George, I know you're a very busy person, and I'm so grateful and thankful that you took time to talk to us today on SAGANet. It's been wonderful having you. Best of luck on your research, and we hope to talk to you again in the future. Those of you who are watching, thank you for your questions. #AskAstrobio. Don't forget to let me know if you know what's behind me, where is that picture taken from. Until then, we'll talk to you all next month, for a new episode of Ask an Astrobiologist. Please stay curious. Bye-bye.
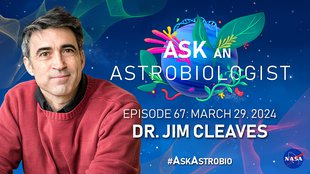
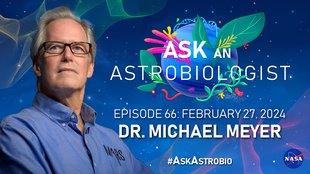
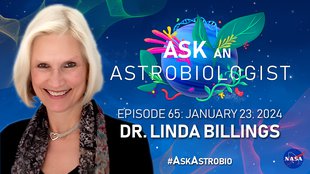
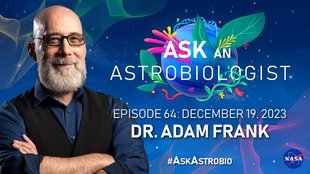
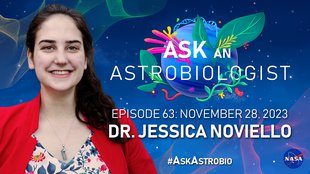
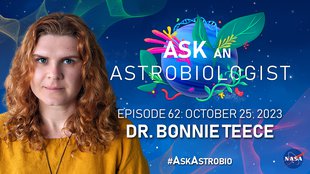
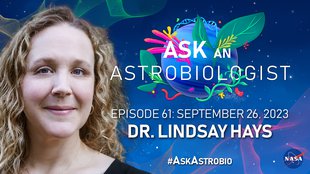
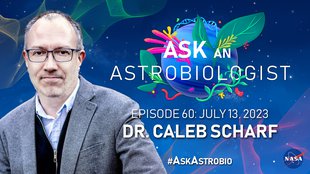
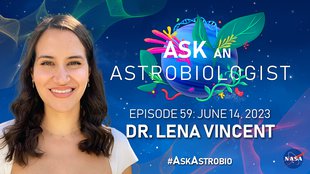
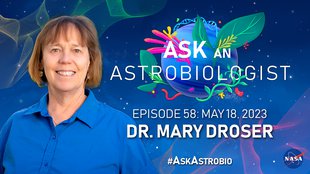
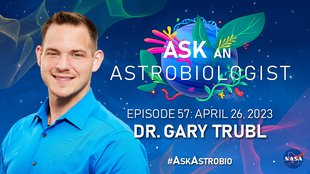
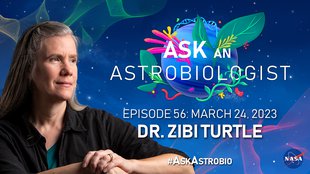
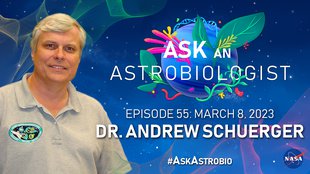
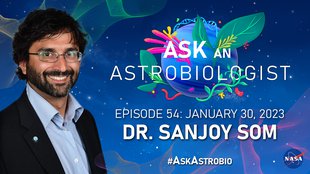
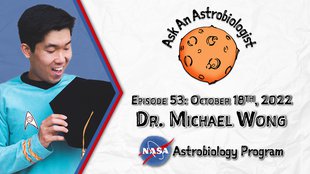
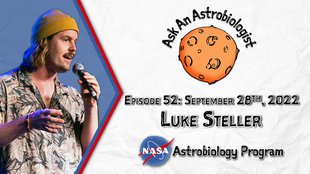
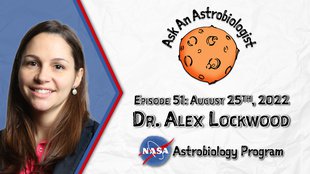
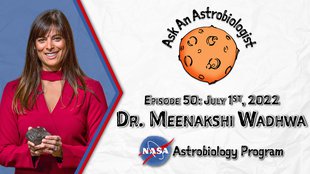
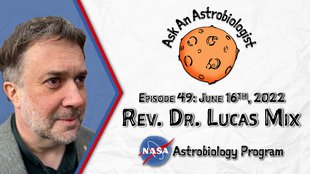
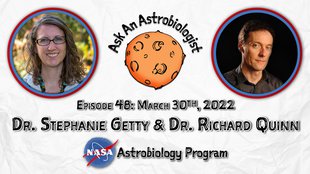
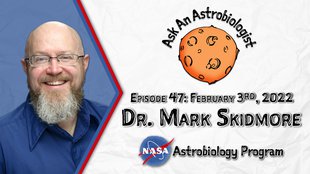
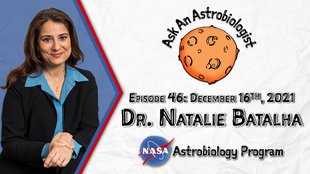
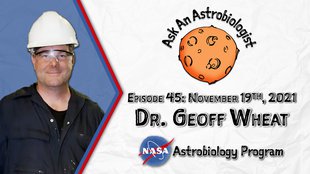
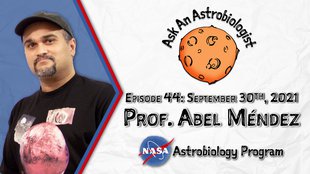
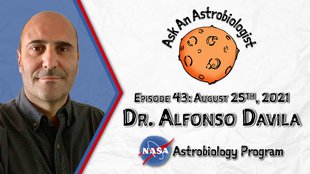
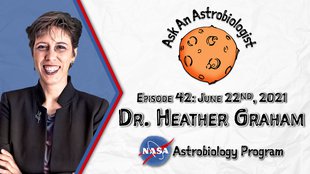
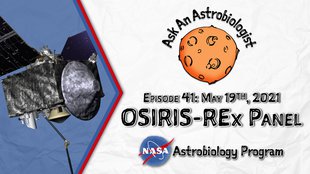
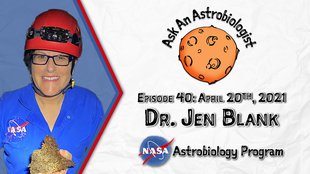
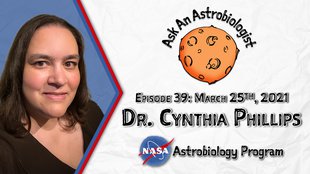
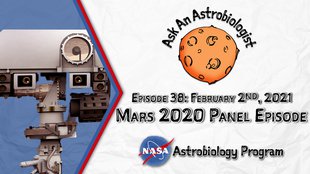
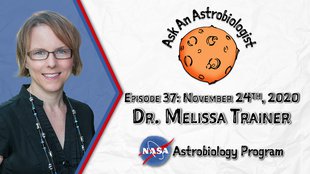
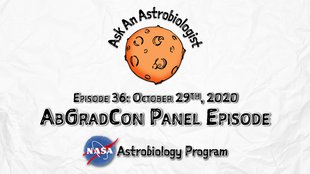
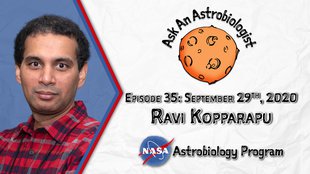
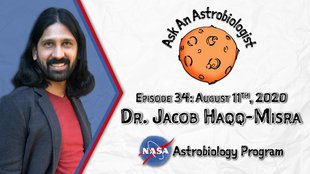
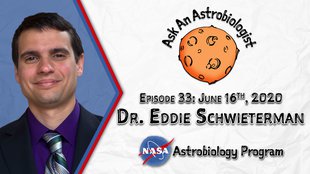
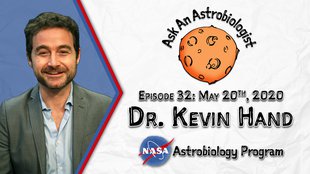
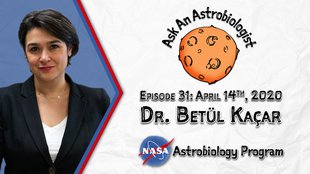
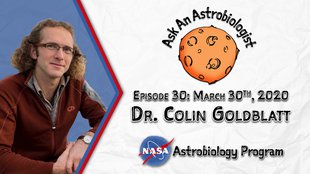
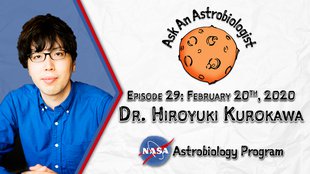
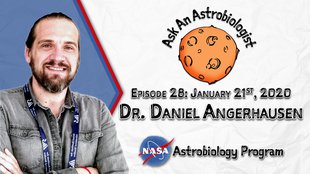
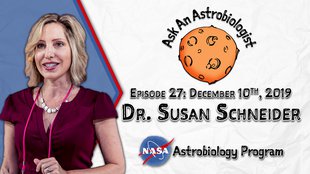
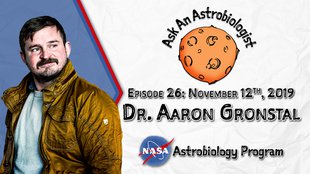
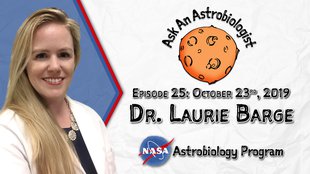
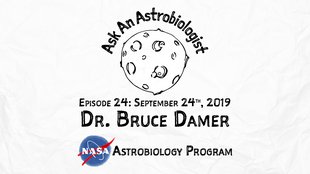
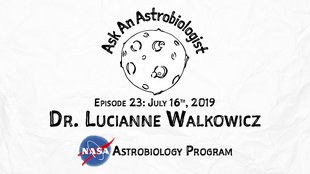
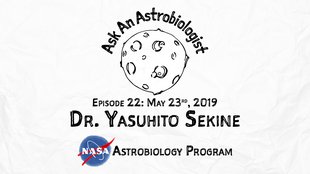
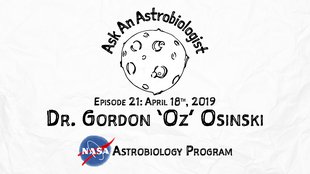
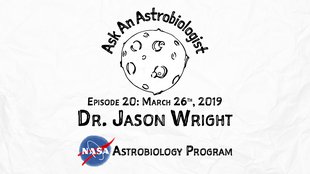
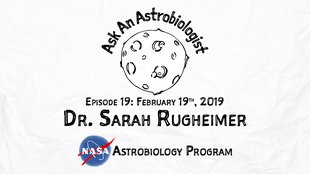
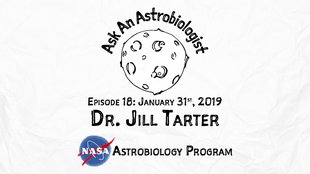
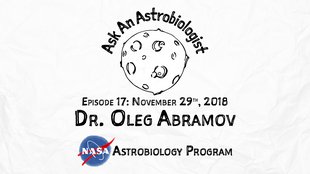
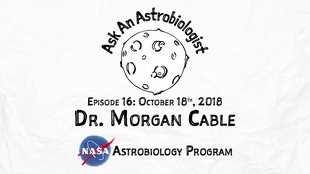
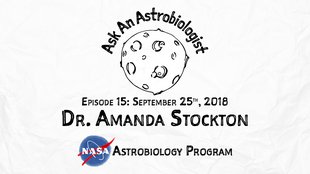
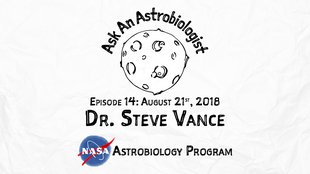
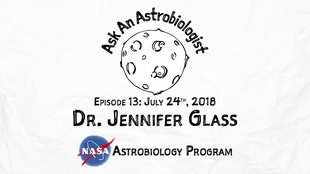
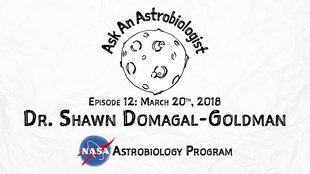
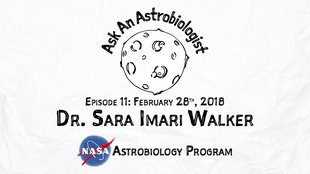
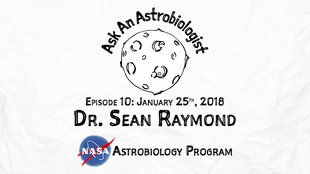
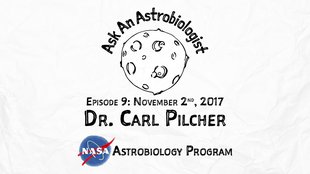

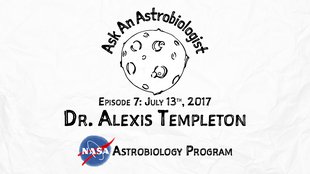
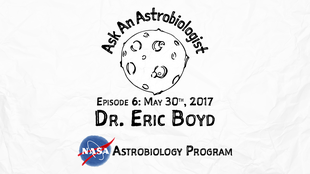
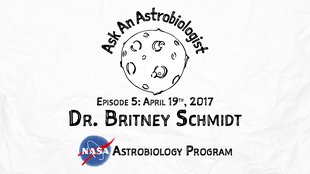
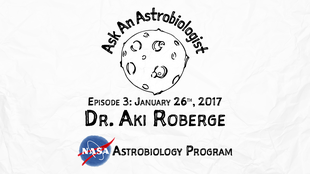
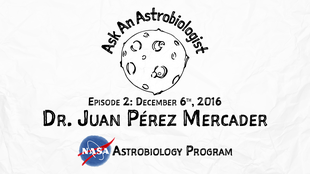
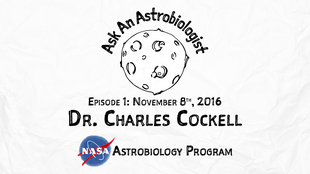