

In trying to tease out what a planet is made of, its density is of great importance. Scientists can use that measure of density — the amount of matter contained in a given volume — to determine what ratio of a planet is likely is gas, or water, or rocks, or rocks and iron and more. They can even help determine if the planet has a central core.
So determining the density of exoplanets is a high priority and one that has been especially important for the Trappist-1 solar system, the amazing collection of seven “Earth-sized” rocky planets orbiting a Red Dwarf star some 40 light years away.
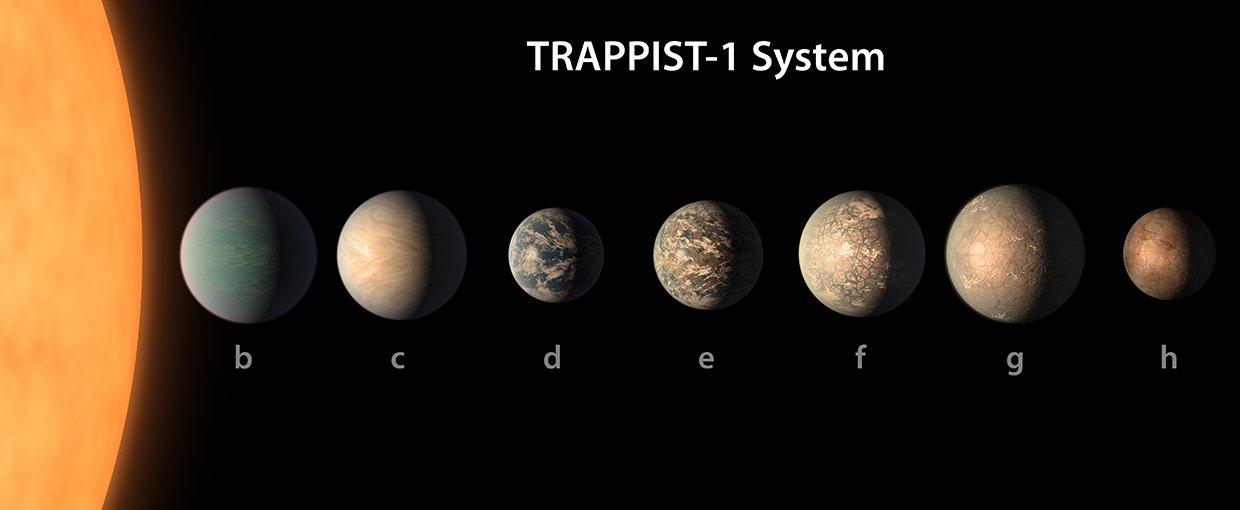
The seven planets of the Trappist-1 solar system. The first planets were discovered five years ago and others in 2017. Trappist-1 is a dream system for researchers to study because it includes so many rocky planets. The planets do, however, orbit very close to a relatively small and cool Red Dwarf star, which makes the system and its potential for habitability different than if they orbited a sun-like star.Image credit: NASA/JPL-Caltech.
The Trappist-1 planets have been a major focus of study since its first planets were discovered in 2016, and now a new and rather surprising finding about the density of the planets has been accepted for publication in the Planetary Science Journal. While the planets are somewhat different sizes, they appear to be all almost the exact same density. This provides a goldmine of information for scientists.
Equally exciting, while the seven Trappist-1 planets have similar densities, they are 8% less dense than they would be if they had the same chemical composition as our planet. It may not seem like a lot, but to astrophysicists it is.
“This is the information we needed to make hypotheses about their composition and understand how these planets differ from the rocky planets in our solar system,” said lead author Eric Agol of the University of Washington.
What Agol considers the team’s most robust conclusions: The Trappist-1 planets have a “common make-up” just as the rocky planets in our solar system do, but are nonetheless in some significant ways different from our rocky planets. “TRAPPIST-1 has a different ‘recipe’ for forming terrestrial planets, and a more uniform recipe as well,” he told me.
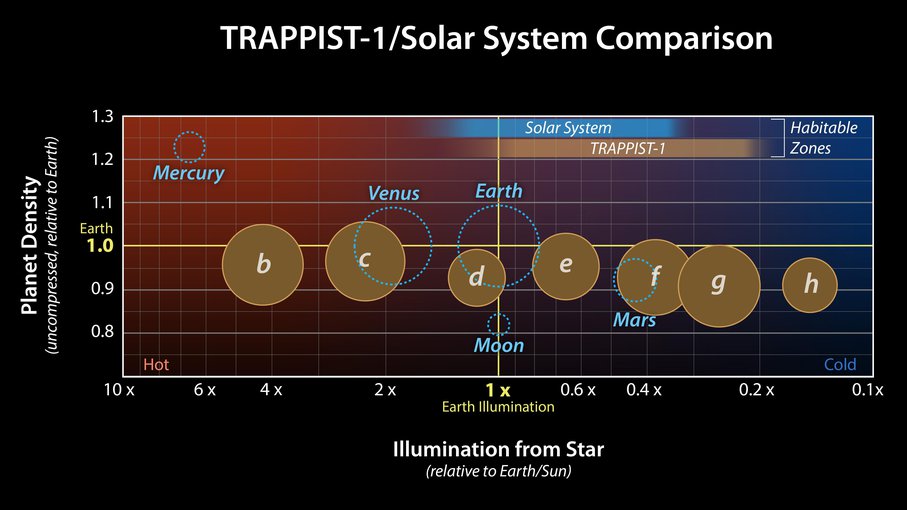
A planet’s density is determined not just by its composition, but also by its size: Gravity compresses the material a planet is made of, increasing the planet’s density. Uncompressed density adjusts for the effect of gravity, and can reveal how the composition of various planets compare.Image credit: NASA/JPL-Caltech.
The team — which includes scientists based in the United States, Switzerland, France, the United Kingdom and Morocco — used observations of the starlight dips and precise measurements of the timing of the planets’ orbits to make their detailed measurements of each planet’s mass and diameter, and from there to determine its density. Agol and UW co-authors Zachary Langford and Victoria Meadows, a professor of astronomy, analyzed data and performed computer simulations that constrained the orbits of the TRAPPIST-1 planets and calculated their densities.
All seven TRAPPIST-1 planets, which are so close to their star that all seven would fit within the orbit of Mercury, were found via the transit method: Scientists can’t see the planets directly (they’re too small and faint relative to the star), so they look for dips in the star’s brightness created when the planets cross in front of it.
Repeated observations of the starlight dips combined with measurements of the timing of the planets’ orbits enabled astronomers to estimate the planets’ masses and diameters, which were in turn used to calculate their densities. Previous calculations determined that the planets are roughly the size and mass of Earth and thus must also be rocky, or terrestrial – as opposed to gas-dominated, like Jupiter and Saturn. The new paper offers the most precise density measurements yet for any group of exoplanets.
The more precisely scientists know a planet’s density, the more limits they can place on its composition. As described in a release: “Consider that a paperweight might be about the same size as a baseball yet is usually much heavier. Together, width and weight reveal each object’s density, and from there it is possible to infer that the baseball is made of something lighter (string and leather) and the paperweight is made of something heavier (usually glass or metal).”
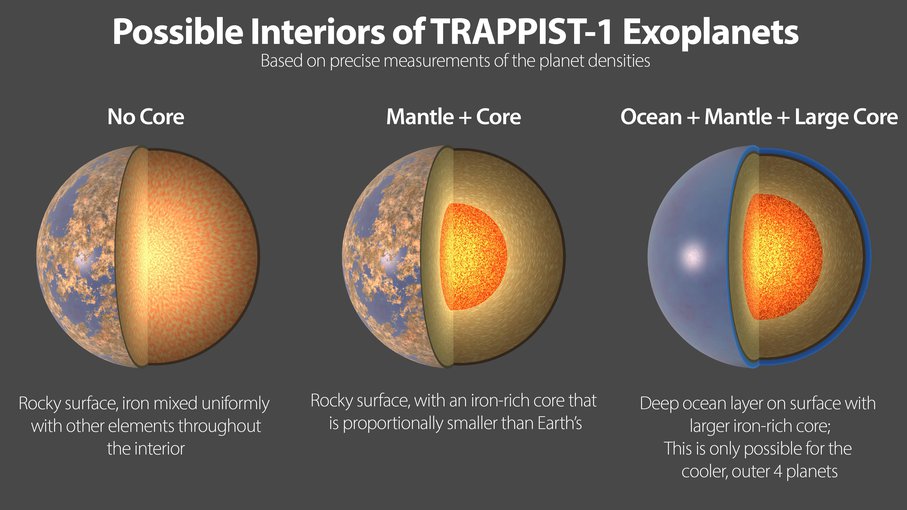
An artist’s depiction of three possible interiors of the TRAPPIST-1 exoplanets. The more precisely scientists know the density of a planet, the more they can narrow down the range of possible interiors for that planet.Image credit: NASA/JPL-Caltech.
In contrast to the Trappist-1 system, the densities of the eight planets in our own solar system vary widely.
The gas-dominated giants – Jupiter, Saturn, Uranus, and Neptune – are larger but much less dense than the four terrestrial worlds because they’re composed mostly of lighter elements like hydrogen and helium. Even the four terrestrial worlds show some variety in their densities, which are determined by both a planet’s composition and compression due to the gravity of the planet itself. By subtracting the effect of gravity, scientists can calculate what’s known as a planet’s uncompressed density and potentially learn more about a planet’s composition.
The difference in density between the TRAPPIST-1 planets and Earth, Venus and Mars, may seem small — that 8% — but it is significant on a planetary scale. For example, one way to explain the lower density is that the TRAPPIST-1 planets have a similar composition to Earth, but with a significantly lower percentage of iron — about 21% compared to Earth’s 32%, according to the study.
Alternatively, the iron in the TRAPPIST-1 planets might be combined with high levels of oxygen, forming iron oxide, or rust. The additional oxygen would decrease the planets’ densities. The surface of Mars gets its red tint from iron oxide, but like its three terrestrial siblings, it has a core composed of non-oxidized iron.
By contrast, if the lower density of the TRAPPIST-1 planets were caused entirely by oxidized iron, then the planets would have to be rusty throughout and could not have iron cores. Agol said the answer might be a combination of the two scenarios — less iron overall and some oxidized iron.
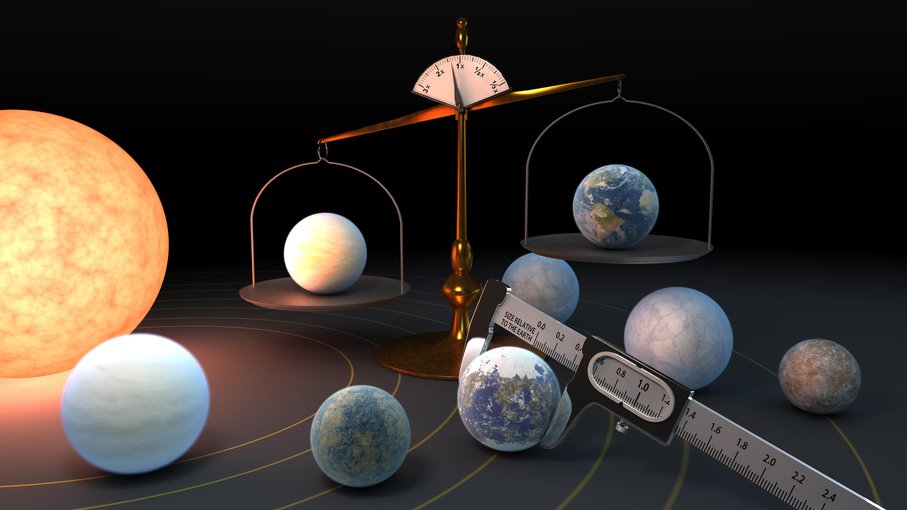
Scientists can determine the rough composition of planets by measuring their size, the pace of their orbits and their density. This can all be done without actually observing the planet but rather measuring their interactions with their host suns.Image credit: NASA/JPL-Caltech.
The take-home message, then, is that basic measurements of exoplanets can help scientists predict what elements may be present on the planets and it what amounts.
We know the abundance by mass of the nine most common elements in the Earth’s crust: approximately oxygen 46%, silicon 28%, aluminum 8.3%, iron 5.6%, calcium 4.2%, sodium 2.5%, magnesium 2.4%, potassium 2.0%, and titanium 0.61%.
Scientists are nowhere near being able to make those kinds of measurements on exoplanets, but they have begun the process.
For instance, Agol explained that a model created by co-author Caroline Dorn of The University of Zurich uses mass and radius measures to show that the Trappist-1 planets may have the same ratios of iron, magnesium and silicon as our sun, which also approximates the ratios on our terrestrial planets. In this model, the Trappist-1 planets do not have a central core — the iron is oxidized and mixed throughout the mantle.
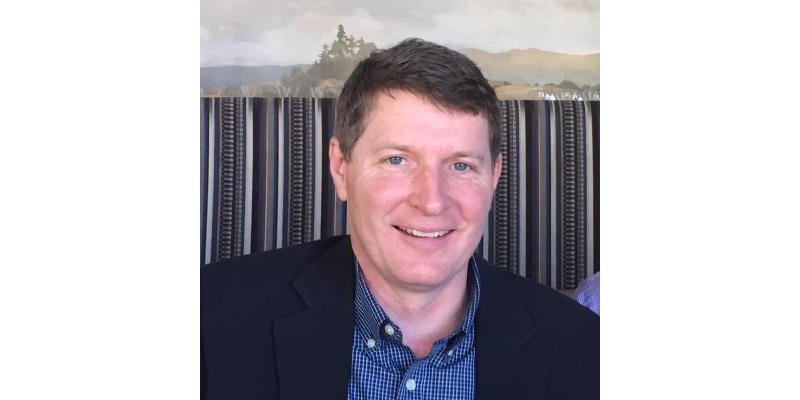
Image credit: Eric Agol / Many Worlds.
(Scientists typically measure the abundance of heavy elements in a star relative to the iron content in its atmosphere, as iron is a common element and its spectrum is relatively easy to measure. )
Speaking of Dorn’s model, Agol said that “What was impressive to me is that without any adjustment of this model, the line goes through all of the high-probability regions for all seven planets! Now, this could just be a coincidence, but it was surprising to me that the core-free model worked so well.”
“The caveat is that we do not know the iron/magnesium/silicon ratio of the TRAPPIST-1 star — it’s a cold star which makes these elements difficult to detect in its atmosphere, And so we don’t know if the ratios of these elements are similar to our sun. This is all to say we cannot draw precise conclusions” with the data available so far.
When it launches, NASA’s James Webb Space Telescope should have the capabilities to probe this system further, including gathering more detailed information about the atmospheres of the seven TRAPPIST-1 worlds.
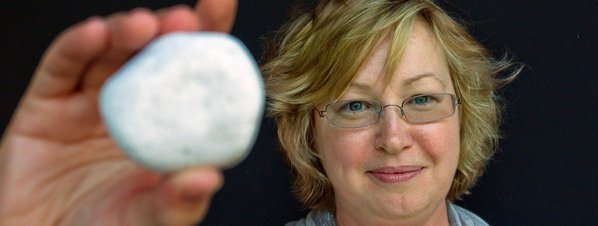
Victoria Meadows is an astrobiologist and planetary astronomer whose research interests focus on acquisition and analysis of remote-sensing observations of planetary atmospheres and surfaces. Since 2000, she has been the Principal Investigator for the pioneering Virtual Planetary Laboratory. She is also a co-lead of NExSS.Image credit: University of Washington.
The team also looked into whether the surface of each planet could be covered with water, which is even lighter than rust and which would change the planet’s overall density. If that were the case, water would have to account for about 5% of the total mass of the outer four planets. By comparison, water makes up less than 0.1% of Earth’s total mass, so this is considered unlikely.
The three inner TRAPPIST-1 planets are too close to their star for water to remain a liquid under most circumstances, would require hot, dense atmospheres like on Venus, where water could remain bound to the planet as steam. But this explanation seems less likely because it would be a coincidence for all seven planets to have just enough water present to have such similar densities, Agol said.
Dorn described the TRAPPIST-1 system as ” fascinating because around this one star we can learn about the diversity of rocky planets within a single system. And we can actually learn more about a planet by studying its neighbors as well, so this system is perfect for that.”
Related:
10 Things: All About TRAPPIST-1 (NASA)
New Clues to TRAPPIST-1 Planet Compositions, Atmospheres (NASA)
What is an Exoplanet? (NASA)
Possible Interiors of the Trappist-1 Exoplanets (NASA)
The Many Worlds Blog chronicles the search for evidence of life beyond Earth written by author/journalist Marc Kaufman. The “Many Worlds” column is supported by the Lunar Planetary Institute/USRA and informed by NASA’s NExSS initiative, a research coordination network supported by the NASA Astrobiology Program. Any opinions expressed are the author’s alone.