2014 Annual Science Report
Arizona State University
Reporting | SEP 2013 – DEC 2014
Stoichiometry of Life, Task 2a: Field Studies - Yellowstone National Park
Project Summary
Yellowstone National Park harbors an array of hydrothermal ecosystems with widely varying geochemical characteristics and microbial communities. Our research aimed to understand how the geochemistry of these hot springs shapes their constituent microbial communities including their composition and function. To accomplish this aim, we measured (1) physical and geochemical properties of hot spring fluids and sediments, (2) the rates of biogeochemical processes (i.e., methane oxidation, nitrogen fixation, microbial Fe cycling, photosynthesis, de-nitrification, etc.), and (3) markers for microbial community diversity (i.e., SSU rRNA, metabolic genes, lipids, proteins).
Project Progress
Tracking Major Nutrients from Geochemistry to Biochemistry – This area is a major focus that links our fieldwork in Yellowstone with lab analyses and interpretations. We are tracking C and N from hot spring fluids and sediments to biomolecules in microbes including lipids, proteins, and pigments. At the same time we are placing these studies in the broader context of C and N cycles involved in energy conservation and material uptake by microbial communities. Finally, much of this work is reflected in the development of some Principles of Geobiochemistry (Shock & Boyd, 2015).
Following the Elements from Hot Spring Fluids to Lipids – Compound-specific stable isotope measurements were used by Schubotz et al. (2015), who built on their lipid results reported in 2013 from chemotrophic microbial communities by focusing on two alkaline springs of the Lower Geyser Basin. This time the emphasis shifted to using stable isotopes in experiments to follow C from the environment into microbial cells. Isotopically labeled bicarbonate, formate, acetate and glucose were used in the experiments. Of these, acetate was the most readily consumed form of carbon in both field locations, indicating that heterotrophy is relative common in these high temperature communities. Uptake of glucose occurred but at much slower rates, and rates of formate uptake were difficult to detect. On the other hand, bicarbonate uptake at one spring and not the other indicates that autotrophy also occurs in these communities. Labeled carbon from acetate was detected in archaeal lipids, suggesting that heterotrophy is a major metabolic strategy for archaea in hot springs. There are major implications in these results for interpreting compound-specific isotopic data from biomarker compounds in the ancient geologic record. This work resulted from a major collaborative effort between Florence Schubotz and Lindsay Hays at MIT and D’Arcy Meyer-Dombard at U. Ill. – Chicago.
Recent results from lipids extracted from a suite of hot springs by ASU graduate student Grayson Boyer and analyzed by Boyer with Flo Schubotz and Roger Summons at MIT show how molecular compositions of lipids, especially bacterial hopane polyols (BHPs) depend on hot spring compositions, suggesting novel uses for the biomarker record. In particular, Boyer has found a strong correlation between the nitrogen content of hot spring fluids and the abundances and varieties of N-bearing BHPs. In parallel he is developing estimation methods for predicting the thermodynamic properties of BHPs. These data will allow independent tests of the relative energetic costs of making different BHPs in diverse environments, and predictions about the energetic changes that accompany BHP alteration from living lipids to the molecules preserved in the geologic record. Results were presented in a poster at the Fall 2014 AGU meeting.
Following the Microbial Carbon Cycle – An example of the intersection of the C cycle with energy uptake is the documentation by Poret-Peterson et al. (now in revision) of methane production and consumption in a small hot spring at the edge of Obsidian Pool by combining stable isotope labeling with gene extraction and enumeration. In particular, we have found an active methane cycle in a slightly acidic hot spring (pH ~6; T=~67°C) using activity assays (tracing the conversion of methane to carbon dioxide) and molecular analyses (detection of transcripts of metabolic genes for methanogenesis and methanotrophy). These results help to quantify how methanotrophic bacteria act as ‘biological filters’ for methane produced by archaea, and emphasize the dynamics of the methane cycle in continental hot springs.
Quantifying the Microbial Iron Cycle – ASU graduate student Brian St. Clair continued his research to actively quantify rates of abiotic and biological iron transformations, with field experiments in Yellowstone and elsewhere. This work, described in prior years’ reports, continues to reveal that the abiotic rate of iron oxidation becomes increasingly rapid with increasing pH eventually creating conditions where biological iron oxidation cannot compete. Similarly, the abiotic rate of iron reduction increases with decreasing pH, such that microbes are unable to compete with the abiotic rate at extremely acidic conditions. The interplay of these abiotic reaction rates creates a zone of microbial habitability for the iron cycle in hot springs between pH values of roughly 1.5 and 5. In most locations, where there is strong visual evidence of iron oxidation (extensive red ferric oxyhydroxide staining) the rate of microbial iron reduction rivals that of microbial oxidation, implying that the geologic accumulation of iron biominerals may result from a slight shift of the redox cycle balance toward oxidation over time. Latest results were presented in a poster at the Fall 2014 AGU meeting.
The Transition to Photosynthesis – The transition to photosynthesis is the single most profound change in hot spring ecosystems. On one side the productivities of microbial communities are limited by the amount of geochemical energy provided by disequilibria, while on the other where sunlight is also used as an energy source community productivities may be limited by nutrient supplies. The multifaceted geochemical and biochemical transitions at the photosynthetic fringe are the focus of coordinated elemental, isotopic, molecular, and modeling studies by Shock and co-workers. The goal is to link the transformations they have begun to document in lipids, proteins, pigments and inorganic compositions along hot spring outflow channels that cross the photosynthetic fringe. Major developments include using metastable equilibrium calculations (Dick & Shock, 2013) to characterize the shift in energy supplies. This year, ASU post-doc Alysia Cox worked to analyze proteins extracted from biofilm samples from either side of the photosynthetic fringe guided by metagenomic data from the same samples, while ASU graduate students Grayson Boyer and Kris Fecteau examined lipids and pigments, respectively, extracted from the same samples. Fecteau continued his examination of the abundance and chemical speciation of Hg, to see if Hg toxicity suppresses the transition to photosynthesis in some locations. Results were reported at the Fall 2014 AGU meeting.
Extended Redfield Ratios: During the 2009 and 2010 field seasons, we collected 200+ sediment and microbial mat samples for determination of the Extended Redfield Ratio (ERR). We have measured C, N, P, and trace metal content of bulk sediments and microbial mat samples collected in 2009. To enable these measurements, we have adapted and published a cell-sediment separation method that conserves the elemental content of microbial cells collected in extreme environments (reported this year in Neveu et al., 2014). Using this method, we have isolated cells from about 40 sediment samples (160 replicates) collected in hot springs of Yellowstone National Park. Results finally were finally synthesized in the past two years. Surprisingly, despite the tremendous physicochemical variability of these environments (T, pH, redox, nutrient concentrations), we found the major (C, N, P) and trace element composition (Ni, Cu, Zn, Mo) to be in the ranges measured in microbes living in more moderate settings (Neveu et al., in review). The measured C:P and N:P ratios in separated biomass were unusually relatively high compared to the canonical Redfield values, but comparable to those seen in suspended materials in P-limited lakes. Because low P concentrations were also measured in the springs sampled, the low P abundances in separated material may reflect a scarcity of P (compared to C and N) in YNP environments. Differences in the relative usage patterns of Ni, Cu, Zn, and Mo by microbial communities may relate to specific metabolic processes at play in hot springs because these four metals are often used as enzyme cofactors. For example, we measured lower Mo content in chemotrophic vs. phototrophic cells. It may be that the cellular demand for Mo in chemotrophic communities is higher, perhaps for N cycling, formate oxidation, or sulfur oxidation, since Mo is present in enzymes key to these pathways. (Mo did not otherwise correlate with T, pH, or any nutrient concentration.) In the broadest sense, the ordinary composition of these extremophiles suggests a common core of organic and inorganic biochemistry that leads to elemental abundances constrained within a common (broad) envelope typical of all microbes, at least for the above elements. This stoichiometric envelope spans several orders of magnitude in abundance for P and trace metals, likely largely due to microbial adaptation to a variety of elemental supply conditions. Relatively constrained elemental distributions may prove a useful means of detecting extant or extinct Earth-like life. Multi-element deviations from mineral background abundances at the spatial scales of cells or microbial mats along with comparison of such elemental patterns against biological elemental ranges that seem robust to much of Earth’s entire environmental spectrum may provide a useful biosignature.
MEMS sensor development. Increasingly, we are reminded that hydrothermal ecosystems are dynamic systems changing spatially and temporally, and that our sampling efforts at discreet times and locations provide snapshot views. Interpreting those snapshots within the framework of system dynamics is enhanced by efforts to sense changes in major variables. Our efforts to map temperature and conductivity changes in a hot spring mixing zone with MEMS (Micro-Electrical Mechanical Systems) sensors (Oiler et al., 2014) leads to a model of how temperature and composition change on a fine spatial scale. This work underpins a new effort to characterize changes in the microbial community across these small-scale variations instigated by ASU graduate student Alta Howells. By combining geochemical sampling and analysis with molecular methods that reveal microbial diversity (SSU rRNA), Howells is determining the factors that establish ecotones in the mixing zone, and how the mixing process defines habitability. Her work, together with the sensor-based framework, was included in a poster for the Fall 2014 AGU meeting.

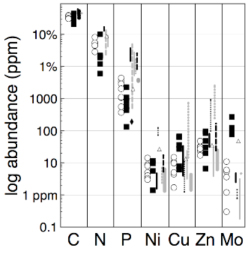
Publications
-
Dick, J. M., & Shock, E. L. (2013). A Metastable Equilibrium Model for the Relative Abundances of Microbial Phyla in a Hot Spring. PLoS ONE, 8(9), e72395. doi:10.1371/journal.pone.0072395
-
Neveu, M., Poret-Peterson, A. T., Lee, Z. M. P., Anbar, A. D., & Elser, J. J. (2014). Prokaryotic cells separated from sediments are suitable for elemental composition analysis. Limnol. Oceanogr. Methods, 12(7), 519–529. doi:10.4319/lom.2014.12.519
-
Oiler, J., Shock, E., Hartnett, H., & Yu, H. (2013). MEMS harsh environment sensor array-enabled hot spring mapping. 2013 IEEE SENSORS. doi:10.1109/icsens.2013.6688332
-
Schubotz, F., Hays, L. E., Meyer-Dombard, D. A. R., Gillespie, A., Shock, E. L., & Summons, R. E. (2015). Stable isotope labeling confirms mixotrophic nature of streamer biofilm communities at alkaline hot springs. Frontiers in Microbiology, 6. doi:10.3389/fmicb.2015.00042
-
PROJECT INVESTIGATORS:
-
PROJECT MEMBERS:
Everett Shock
Project Investigator
Ariel Anbar
Co-Investigator
James Elser
Co-Investigator
Hilairy Hartnett
Co-Investigator
Eric Boyd
Collaborator
Grayson Boyer
Collaborator
Jeffrey Dick
Collaborator
Kristopher Fecteau
Collaborator
Marc Neveu
Collaborator
Jon Oiler
Collaborator
Amisha Poret-Peterson
Collaborator
Florence Schubotz
Collaborator
Brian St Clair
Collaborator
Roger Summons
Collaborator
-
RELATED OBJECTIVES:
Objective 5.1
Environment-dependent, molecular evolution in microorganisms
Objective 5.2
Co-evolution of microbial communities
Objective 5.3
Biochemical adaptation to extreme environments
Objective 6.1
Effects of environmental changes on microbial ecosystems
Objective 6.2
Adaptation and evolution of life beyond Earth
Objective 7.2
Biosignatures to be sought in nearby planetary systems