2011 Annual Science Report
NASA Ames Research Center
Reporting | SEP 2010 – AUG 2011
Executive Summary
The Ames Team investigates the physical, chemical and biological processes that combined to create and shape early habitable environments. We trace the cosmic evolution of organic molecules from the interstellar medium, through protoplanetary disks and planetesimals, and ultimately to potentially habitable planets. We characterize the diversity of planetary systems that might emerge from protoplanetary disks. We identify diverse scenarios for the origins and early evolution of catalytic functionality and metabolic reaction networks. We develop and test a methodology for assessing quantitatively the habitability of early planetary environments – particularly Mars – via capabilities that could be deployed in situ. Our ongoing active involvement in multiple NASA missions provides context, incentives and collaborative opportunities for our research and education and public outreach programs. Please visit http://www.amesteam.arc.nasa.gov/.
Astrochemistry. We document molecular species in space that are interesting from a biogenic perspective and we seek to understand their possible roles in the origins of life. We achieve this by documenting the spectra and chemistry of analog materials in the laboratory, by conducting remote sensing in small spacecraft and by analyzing extraterrestrial samples that have been returned by spacecraft or that fall to Earth as meteorites. We use these results to interpret astronomical observations.
FIGURE 1. The PAH database has been used to unravel different aromatic components in the reflection nebula NGC 7023. Visible picture shown in Fig.2. Left: Spectra of PAH clusters (red), neutral PAHs (green) and PAH cations (blue) in the ISOCAM spectral-spatial maps of reflection nebula NGC 7023. Right: These components show different spatial distributions in this source reflecting the photo-fragmentation of PAH clusters, followed by the ionization of the free-flying PAHs as they approach the central star (indicated as a white dot).
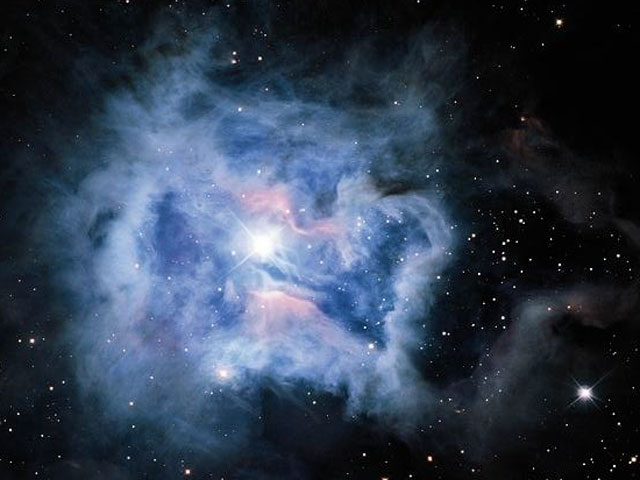
FIGURE 2. Visible image of reflection nebula NGC 7023. The pinkish region above the star coincides with the PAH cluster distribution shown in red in Figure 1. Visible image courtesy of Dr. Adolf Witt.
Previously we had launched a website that allowed the research community to search and utilize our extensive spectroscopic database of polycyclic aromatic hydrocarbons (PAH). This year has been fruitful for utilizing the database. We published the following three papers that support missions such as Spitzer, SOFIA, Herschel, and JWST: a) a thorough analysis of PAH Far-IR spectra, b) a description of how one can use the database and web tools to fit Spitzer spectra, and c) an analysis of PAH evolution across an extended astronomical object, probing its changing conditions (Figs. 1 and 2). We have also added spectra of very large PAHs, ranging in size from C150 to C384, to the database. As of October 1, 2011, 10,845 visitors from 74 countries have accessed and utilized this website, and at least five separate research groups are using its most advanced tools. We published in Astrobiology a paper that describes the production of prebiotic compounds by UV irradiation of cosmic ices. This paper demonstrated that the photolysis of pyrimidine in H2O ices produces a host of new compounds, including the nucleobase uracil. A second paper, now submitted, shows that the addition of ammonia to the ice results in the production of the nucleobase cytosine.
Evolution of protoplanetary disks. We explore evolving protoplanetary disks to understand the formation of primitive planetary bodies that ultimately could host habitable environments. The disk is in many ways an astrochemical “primeval soup” in which abundant elements are assembled into increasingly complex organic compounds and mixed in the disk’s dust and gas envelope.
We published a model of the optical properties of non-spherical and/or inhomogeneous particles as realistic analogs of astrophysical dust grains. For three classes of grains (aggregates, angular irregular and spherical with iron inclusions), scattering and absorption efficiencies of depend strongly on wavelength and grain-shape. Our results will likely yield dust opacities that differ significantly from current estimates, which assume homogeneous spherical grains.
We inferred the surface density distribution of transition disks (disks with inner dust opacity holes within a few AU of the star) and the likely presence of planets. Using gas line observations, we find that massive planets can dynamically carve inner holes in the disk. In other cases, photoevaporation first enhances disk dispersal and creates a gap; viscous accretion then forms an inner hole. The growth and settling of dust grains within a coagulation/fragmentation equilibrium framework do not affect disk dispersal times significantly unless a substantial fraction of solids is sequestered in large (>10 m diameter) objects.
We submitted a new model that locates water ice in protoplanetary disks and that accounts for the evolution of the Sun (luminosity and temperature) over the ~106 years when the disk is active (Fig. 3). The location of the water ice is represented by a two-branched line with a cusp that defines the innermost location of the ice. Because this cusped region overlays the region where gas occurs and terrestrial planets form, precipitation and/or vertical diffusion might enhance the capture of important volatile elements by accreting planets. These concepts are being extended to include habitable zones in extrasolar planetary systems.
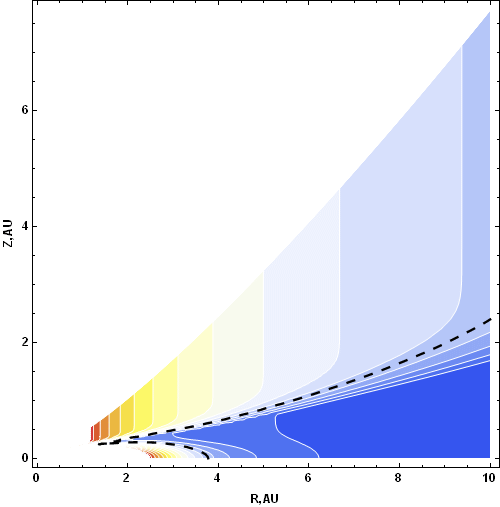
FIGURE 3. Computation of the two-branched ice line in a protoplanetary solar disk superimposed on temperature contours. Note the “viscously heated pocket” to ~4 AU and the radiatively-heated outer branch. The two curves meet at a cusp that defines the closest point of water ice to the central star.
Water and climates. We showed that dry, desert planets have wider habitable zones than water-rich ocean worlds. When an ocean world is close to a star it is more susceptible to the runaway greenhouse effect, whereas a desert planet maintains habitable polar caps separated by an extremely hot tropical belt. An ocean world far from a sun suffers an ice-albedo catastrophe in which the oceans freeze over and the surface temperature falls to below 200 K. A dry planet hasn’t enough water to develop global ice cover, thus its albedo is lower and its tropics remain habitable, albeit rather cold.
Exoplanetary systems data analysis. We led the development of the publicly available Systemic Console software that analyzes radial velocity and photometric data for extrasolar planets. We implemented a programming interface that allows users to create Monte-Carlo simulations. We published a correlation between the metallicity of a star and the occurrence of low-mass planets orbiting low-mass stars observed by the Kepler mission. In another paper we used data from the Kepler mission and the Geneva HARPS Doppler radial velocity survey to show that the density distribution of low-mass short-period planets in the galaxy is likely to be strongly bimodal.
Origins of functional proteins. The origins and early evolution of functional proteins and metabolic reaction networks are essential for biological complexity to emerge in early habitable environments. We are investigating whether protein functionality can arise from an inventory of polymers with amino acid sequences that might have naturally existed in habitable environments. We attempt the first demonstration of multiple origins of a single enzymatic function, and we investigate experimentally how primordial proteins could evolve through the diversification of their structure and function. Building on this work and on our knowledge of ubiquitous protocellular functions and constraints of prebiotic chemistry, we conduct computer simulations aimed at elucidating fundamental principles that govern coupled evolution of early metabolic reactions, their catalysts and transport across cell walls.
We refined the structure of the artificial RNA ligase enzyme, which was isolated from a randomized non-catalytic library of polypeptides. We showed that this enzyme exhibits a fold and catalyses a reaction not existing in nature. Even though the protein is catalytically active, its structured part is quite small and the rest of the protein consists of a flexible loop. This architecture is at variance with the basic paradigm developed for modern enzymes, which requires that functional proteins are compact. This result demonstrates that primordial enzymes, which did not undergo fine-tuning through billions of years of evolution, were different from contemporary enzymes. To probe the proposed zinc-binding sites in our enzyme (Fig. 4a), we individually replaced amino acids that could potentially coordinate zinc and tested the mutants for activity and secondary structure changes. Data on 30 mutants and titration of the enzyme with the substrate combined with the measurements of NMR loss of signal (Fig. 4b) helped to identify the potential substrate-binding site.
FIGURE 4. NMR structure of artificial RNA ligase enzyme. (a) The protein structure contains two zinc ions (displayed as balls) that are likely bound by the zinc-coordinating amino acids shown as stick models. (b) The potential substrate-binding region (red box) was identified by NMR while mixing protein and substrate. The signal intensity symbolizes the degree of change in NMR signal upon substrate binding.
We are also investigating the possibility that the same catalytic ligase activity could emerge from entirely random sequences. We have optimized the ligase selection procedure for a protein library containing 80 random amino acids and are poised to begin the selection.
In order to explore how ancestral proteins evolved to acquire new functions we redesigned computationally an adenosine triphosphate (ATP)-binding protein, previously evolved from random sequences of amino acids, to bind guanosine triphosphate (GTP). The redesign involved not only changing the orientation of the loop that hydrogen bonds to the nucleobase but also structural modifications that stabilize the binding pocket and prevent water from accessing the binding site. We will study the activity of the redesigned protein in the laboratory. We will also explore whether protein evolution from binding ATP to binding GTP can proceed without loss of function.
Quantifying the potential for habitability. Our goal is to discern the habitability (potential to support life) of ancient Martian environments, in particular to discern which environments could have supported more life than others. This information will help to guide the selection of landing sites for missions that seek direct evidence of life or prebiotic evolution. Our approach is two-fold. We use the presence of specific minerals or groups of minerals – an analysis that can be performed robotically on Mars — to constrain the chemical and physical conditions of the ancient environments in which they formed. We characterize the distribution of life on Earth in a series of environments spanning those same parameters, in order to inform the first portion of the investigation.
We conducted field operations at the McLaughlin Natural Reserve (Fig. 5), which offers an important venue for understanding the biological potential of serpentinizing systems. The site differs substantially from many previously studied examples of serpentinizing systems and thus broadens our understanding of the relationship between biology and serpentinized rocks/fluids. The site allows access to subsurface communities through drilling and time-resolved down-hole experimentation. We characterized the chemistry, microbial community structure and metabolic activity of waters collected from existing wells that penetrate the serpentinizing zone. We found waters that clearly exhibit evidence of serpentinization (high pH and low dissolved inorganic carbon) but that had considerably lower dissolved H2 levels than observed at research sites elsewhere. The structure of the microbial communities was generally comparable to those in spring effluents from other geographically diverse serpentinizing systems. We documented biological consumption of both H2 and CO in waters with pH values up to 11.5.
We then conducted a two-week drilling project to collect cores and establish wells at a variety of depths and targeted sites within the McLaughlin reserve. We retrieved one core from each of two sites, to depths of 125-150 feet. We drilled a series of satellite wells (no core retrieval) adjacent to the primary holes, at depths determined by our preliminary core analyses. These wells tapped actively serpentinizing zones that exhibited pH values up to 11.7 and super-saturated methane. We archived the core materials and also collected depth-resolved samples for analysis of microbial community structure (DNA and cDNA libraries, and metagenomic content), organic geochemistry, and mineralogy. The established wells will be monitored in time course for aqueous chemistry, and will be used for down-hole deployment of microbial growth experiments. Initial experiments (using methodology identical to that described for the established wells) did not show biological consumption of H2 or CO, possibly commensurate with the limited energy available in these metabolisms in the horizons accessed by drilling.
FIGURE 5. Drilling operations at the McLaughlin Natural Reserve.
We utilized samples collected from our Josephine Ophiolite locality (near the CA/OR border) to conduct comparative analyses using instruments comparable to the MSL ChemCam and CheMin instruments, as a means of informing Mars surface operations by ground-truthing against well studied terrestrial locales. We deployed a prototype hybrid Moessbauer-XRD instrument at our Hawaiian field site. The prototype could lead to an instrument on future Mars missions that can assess mineral suites as potential indicators of past habitable environments.
Ames Team members pursued activities in education and public outreach (EPO) that included efforts in high-impact public venues. Team research efforts have contributed source material for the team’s EPO program. We maintain a graduate-level astrobiology course at the University of California at Santa Cruz. We provided lab tours and demonstrations to students and educators. We delivered lectures to K-12 classrooms, universities, museums, planetariums, national park visitor centers, scientific conferences and the public media. The Team continued to forge its strong partnership with Lassen Volcanic National Park by exploring astrobiological aspects of hydrothermal activity and microbiology. Ames team members collaborated with staff at Red Bluff High School and Lassen Park to offer the Lassen Astrobiology Student Intern Program to high school juniors and seniors. Interns examined hydrothermal features, collected field data (Fig. 6) and generated a database of temperature, pH, GPS coordinates, photos, and geochemical and biological analyses. They presented their final report to the Red Bluff community. Their report will enrich astrobiological research, classroom lectures, and public talks. Ames team members continue to work with Lassen Park interpretive staff to develop trailside signs that will be located at sites that illustrate astrobiology research at Lassen. Park staff installed the first in a series of all-weather trailside signs.
FIGURE 6. Lassen Astrobiology Student Interns measure the temperature and pH of an acidic, iron-rich spring at Lassen Volcanic National Park.
The NAI Ames Team maintained its substantial presence in current and upcoming NASA missions. The P.I. is a strategic planning lead for the Mars Exploration Rover mission and a member of the Mars Reconnaissance Orbiter CRISM team and the Mars Science Laboratory (MSL) mission CheMin team. The P.I. is also Chair of MEPAG (NASA’s Mars Exploration Program Analysis Group). An Ames Team Co-I is P.I. of the CheMin X-ray diffraction spectrometer for the MSL mission. Other team members participate in the following ongoing or future missions: Kepler, Stardust, Stratospheric Observer for Infrared Astronomy, Mars Reconnaissance Orbiter, MSL, James Webb Space Telescope, Spitzer Space Telescope, Herschel, O/OREOS, ABE/ASPIRE, LCROSS, LADEE, and the Comet Coma Rendezvous Sample Return mission.
Publications
-
Abe, Y., Abe-Ouchi, A., Sleep, N. H., & Zahnle, K. J. (2011). Habitable Zone Limits for Dry Planets. Astrobiology, 11(5), 443–460. doi:10.1089/ast.2010.0545
-
Allen, M. A., Neilan, B. A., Burns, B. P., Jahnke, L. L., & Summons, R. E. (2010). Lipid biomarkers in Hamelin Pool microbial mats and stromatolites. Organic Geochemistry, 41(11), 1207–1218. doi:10.1016/j.orggeochem.2010.07.007
-
Alperin, M., & Hoehler, T. (2010). The Ongoing Mystery of Sea-Floor Methane. Science, 329(5989), 288–289. doi:10.1126/science.1189966
-
Anderson, R. B., Morris, R. V., Clegg, S. M., Bell, J. F., Wiens, R. C., Humphries, S. D., … McInroy, R. (2011). The influence of multivariate analysis methods and target grain size on the accuracy of remote quantitative chemical analysis of rocks using laser induced breakdown spectroscopy. Icarus, 215(2), 608–627. doi:10.1016/j.icarus.2011.07.034
-
Bera, P. P., Nuevo, M., Milam, S. N., Sandford, S. A., & Lee, T. J. (2010). Mechanism for the abiotic synthesis of uracil via UV-induced oxidation of pyrimidine in pure H[sub 2]O ices under astrophysical conditions. J. Chem. Phys., 133(10), 104303. doi:10.1063/1.3478524
-
Boersma, C., Bauschlicher, C. W., Ricca, A., Mattioda, A. L., Peeters, E., Tielens, A. G. G. M., & Allamandola, L. J. (2011). POLYCYCLIC AROMATIC HYDROCARBON FAR-INFRARED SPECTROSCOPY. The Astrophysical Journal, 729(1), 64. doi:10.1088/0004-637x/729/1/64
-
Bouwman, J., Cuppen, H. M., Steglich, M., Allamandola, L. J., & Linnartz, H. (2011). Photochemistry of polycyclic aromatic hydrocarbons in cosmic water ice. A&A, 529, A46. doi:10.1051/0004-6361/201015762
-
Bouwman, J., Mattioda, A. L., Linnartz, H., & Allamandola, L. J. (2010). Photochemistry of polycyclic aromatic hydrocarbons in cosmic water ice. A&A, 525, A93. doi:10.1051/0004-6361/201015059
-
Chiar, J. E., Pendleton, Y. J., Allamandola, L. J., Boogert, A. C. A., Ennico, K., Greene, T. P., … Eriksson, K. (2011). ICES IN THE QUIESCENT IC 5146 DENSE CLOUD. The Astrophysical Journal, 731(1), 9. doi:10.1088/0004-637x/731/1/9
-
Clemett, S. J., Sandford, S. A., Nakamura-Messenger, K., HÖRZ, F., & McKAY, D. S. (2010). Complex aromatic hydrocarbons in Stardust samples collected from comet 81P/Wild 2. Meteoritics & Planetary Science, 45(5), 701–722. doi:10.1111/j.1945-5100.2010.01062.x
-
Crumpler, L. S., Arvidson, R. E., Squyres, S. W., McCoy, T., Yingst, A., Ruff, S., … Hurowitz, J. (2011). Field reconnaissance geologic mapping of the Columbia Hills, Mars, based on Mars Exploration Rover Spirit and MRO HiRISE observations. Journal of Geophysical Research, 116. doi:10.1029/2010je003749
-
Geers, V. C., Gorti, U., Meyer, M. R., Mamajek, E., Benz, A. O., & Hollenbach, D. (2012). REMNANT GAS IN EVOLVED CIRCUMSTELLAR DISKS: HERSCHEL PACS OBSERVATIONS of 10-100 Myr OLD DISK SYSTEMS. The Astrophysical Journal, 755(1), 8. doi:10.1088/0004-637x/755/1/8
-
Gorti, U., Hollenbach, D., Najita, J., & Pascucci, I. (2011). EMISSION LINES FROM THE GAS DISK AROUND TW HYDRA AND THE ORIGIN OF THE INNER HOLE. The Astrophysical Journal, 735(2), 90. doi:10.1088/0004-637x/735/2/90
-
Hoehler, T. M., & Westall, F. (2010). Mars Exploration Program Analysis Group Goal One: Determine If Life Ever Arose on Mars. Astrobiology, 10(9), 859–867. doi:10.1089/ast.2010.0527
-
Lissauer, J. J., Fabrycky, D. C., Ford, E. B., Borucki, W. J., Fressin, F., Marcy, G. W., … Steffen, J. H. (2011). A closely packed system of low-mass, low-density planets transiting Kepler-11. Nature, 470(7332), 53–58. doi:10.1038/nature09760
-
Nakamura, T., Noguchi, T., Tanaka, M., Zolensky, M. E., Kimura, M., Tsuchiyama, A., … Kawaguchi, J. (2011). Itokawa Dust Particles: A Direct Link Between S-Type Asteroids and Ordinary Chondrites. Science, 333(6046), 1113–1116. doi:10.1126/science.1207758
-
Nuevo, M., Milam, S. N., Sandford, S. A., De Gregorio, B. T., Cody, G. D., & Kilcoyne, A. L. D. (2011). XANES analysis of organic residues produced from the UV irradiation of astrophysical ice analogs. Advances in Space Research, 48(6), 1126–1135. doi:10.1016/j.asr.2011.05.020
-
Orphan, V. J., & Hoehler, T. M. (2011). Microbiology: Hydrogen for dinner. Nature, 476(7359), 154–155. doi:10.1038/476154a
-
Pascucci, I., Sterzik, M., Alexander, R. D., Alencar, S. H. P., Gorti, U., Hollenbach, D., … Edwards, S. (2011). THE PHOTOEVAPORATIVE WIND FROM THE DISK OF TW Hya. The Astrophysical Journal, 736(1), 13. doi:10.1088/0004-637x/736/1/13
-
Ricca, A., Bauschlicher, C. W., & Allamandola, L. J. (2011). PROTONATED POLYCYCLIC AROMATIC HYDROCARBONS REVISITED. The Astrophysical Journal, 727(2), 128. doi:10.1088/0004-637x/727/2/128
-
Ricca, A., Bauschlicher, J. ,. C. W., & Allamandola, L. J. (2011). THE INFRARED SPECTROSCOPY OF POLYCYCLIC AROMATIC HYDROCARBONS WITH FIVE- AND SEVEN-MEMBERED FUSED RING DEFECTS. The Astrophysical Journal, 729(2), 94. doi:10.1088/0004-637x/729/2/94
-
Richard, D. T., Glenar, D. A., Stubbs, T. J., Davis, S. S., & Colaprete, A. (2011). Light scattering by complex particles in the Moon’s exosphere: Toward a taxonomy of models for the realistic simulation of the scattering behavior of lunar dust. Planetary and Space Science, 59(14), 1804–1814. doi:10.1016/j.pss.2011.01.003
-
Rosenberg, M. J. F., Berné, O., Boersma, C., Allamandola, L. J., & Tielens, A. G. G. M. (2012). Coupled blind signal separation and spectroscopic database fitting of the mid-infrared PAH features (Corrigendum). A&A, 537, C5. doi:10.1051/0004-6361/201016340e
-
Sandford, S. A., Milam, S. N., Nuevo, M., Jenniskens, P., & Shaddad, M. H. (2010). The mid-infrared transmission spectra of multiple stones from the Almahata Sitta meteorite. Meteoritics & Planetary Science, 45(10-11), 1821–1835. doi:10.1111/j.1945-5100.2010.001096.x
-
Schlaufman, K. C., & Laughlin, G. (2011). KEPLER EXOPLANET CANDIDATE HOST STARS ARE PREFERENTIALLY METAL RICH. The Astrophysical Journal, 738(2), 177. doi:10.1088/0004-637x/738/2/177
-
Schroder, C., Klingelhofer, G., Morris, R. V., Bernhardt, B., Blumers, M., Fleischer, I., … De Souza, P. A. (2011). Field-portable Mossbauer spectroscopy on Earth, the Moon, Mars, and beyond. Geochemistry: Exploration, Environment, Analysis, 11(2), 129–143. doi:10.1144/1467-7873/09-iags-018
-
Seelig, B. (2011). mRNA display for the selection and evolution of enzymes from in vitro-translated protein libraries. Nature Protocols, 6(4), 540–552. doi:10.1038/nprot.2011.312
-
Treiman, A. H., & Essene, E. J. (2011). Chemical composition of magnetite in Martian meteorite ALH 84001: Revised appraisal from thermochemistry of phases in Fe–Mg–C–O. Geochimica et Cosmochimica Acta, 75(18), 5324–5335. doi:10.1016/j.gca.2011.06.038
-
Tsuchiyama, A., Uesugi, M., Matsushima, T., Michikami, T., Kadono, T., Nakamura, T., … Kawaguchi, J. (2011). Three-Dimensional Structure of Hayabusa Samples: Origin and Evolution of Itokawa Regolith. Science, 333(6046), 1125–1128. doi:10.1126/science.1207807
-
Vogel, M. B., Des Marais, D. J., Parenteau, M. N., Jahnke, L. L., Turk, K. A., & Kubo, M. D. Y. (2010). Biological influences on modern sulfates: Textures and composition of gypsum deposits from Guerrero Negro, Baja California Sur, Mexico. Sedimentary Geology, 223(3-4), 265–280. doi:10.1016/j.sedgeo.2009.11.013
-
Wei, C., & Pohorille, A. (2011). Permeation of Nucleosides through Lipid Bilayers. J. Phys. Chem. B, 115(13), 3681–3688. doi:10.1021/jp112104r
-
Wilson, M. A., Wei, C., Bjelkmar, P., Wallace, B. A., & Pohorille, A. (2011). Molecular Dynamics Simulation of the Antiamoebin Ion Channel: Linking Structure and Conductance. Biophysical Journal, 100(10), 2394–2402. doi:10.1016/j.bpj.2011.03.054
-
Zolensky, M., Herrin, J., Mikouchi, T., Ohsumi, K., Friedrich, J., Steele, A., … Shaddad, M. H. (2010). Mineralogy and petrography of the Almahata Sitta ureilite. Meteoritics & Planetary Science, 45(10-11), 1618–1637. doi:10.1111/j.1945-5100.2010.01128.x
- , H.E.F. & Team, A. (2011). A terrestrial analogue from Spitsbergen (Svalbard, Norway) for the Comanche carbonate at Gusev Crater. Mars. Lunar Planetary Science 42.
- Achilles, C.N., Ming, D.W., Morris, R.V. & Blake, D.F. (2011). Detection limit of smectite by the CheMin IV laboratory instrument: Preliminary implications for CheMin on the Mars Science Laboratory Mission. LPSC, 42.
- Allamandola, L.J. PAHs and Astrobiology. In: Joblin, C. & Tielens, A.G.G.M. (Eds.). PAHs and the Universe. Vol. EAS Publications Series, 46.
- Amundsen, H.E.F., Benning, L., Blake, D.F., Fogel, M., Ming, D., Skidmore, M., Steele, A. & Team, M. (2011). Cryogenic origin for Mars analog carbonates in the Bockfjord volcanic Complex. LPSC 42. Svalbard Norway.
- Anderson, R.B., Morris, R.V., Clegg, S.M., Bell III, J.F., Humphries, S.D. & Wiens, R.C. (2011). A comparison of multivariate and pre-processing methods for quantitative laser-induced breakdown spectroscopy of geologic samples. Lunar Planet. Sci., XLII.
- Blake, D.F., Treiman, A.H., Morris, R.H., Bish, D.A., H.E.F, A., Steele, A. & Team, A. (2011). Carbonate cements from the Sverrefjell and Sigurdfjell volcanoes. Lunar Planetary Science 42. Svalbard Norway.
- Cardace, D. & Hoehler, T.M. Extremophiles in serpentinizing systems. In: Rajakaruna, H.a. (Eds.). Serpentine: A Model for Evolution and Ecology. University of California Press.
- Cardace, D., Meyer-Dombard, D.R., Hoehler, T.M. & Uzunlar, N. (2010). Complex serpentinizing systems and the deep biosphere: metabolic opportunities depend on the geochemistry of mixing waters. AGU. San Francisco, CA.
- Des Marais, D.J. (2010). Exploring Mars for evidence of habitable environments and life. Proc. Amer. Phil. Soc, 154: 402-421.
- Des Marais, D.J. (2010). Formation and preservation of evidence for habitable environments on Mars. 242nd American Chemical Society Annual Meeting. Denver, CO.
- Des Marais, D.J. (20101). The new Mars: Astrobiology of a neighbor planet. GSA Annual Meeting & Exposition. Denver, CO.
- Ehlmann, B.L., Cardace, D., Hoehler, T., Blake, D. & Kelemen, P. (2011). Terrestrial serpentinizing systems as mineralogical, geochemical (and biological?) analogs for Mars. Analogue sites for Mars missions: MSL and beyond. The Woodlands, TX.
- Golden, D.C., Koster, A.M., Ming, D.W., Morris, M.S.A. & Graff, T.G. (2011). Experimental acid weathering of Fe-bearing Mars analog minerals and rocks: Implications for aqueous origin of hematite-bearing sediments in Meridiani Planum. Mars, Lunar Planet. Sci., XLII.
- Gorti, U. (2010). Gas Emission and Origin of the Hole in the TW Hya Disk. Herschel Space Observatory meeting. Goteburg, Sweden.
- Gorti, U. (2011). SPICA and Gas in Circumstellar Disks. 218th Annual Meeting of the American Astronomical Society. Boston.
- Gorti, U. (2011). Emission Lines from Gas in Circumstellar Disks. SOFIA Colloquium series. NASA Ames Research Center.
- Gorti, U. (2011). Gas Emission from Protoplanetary Disks. BAAS, 218(314).
- Gorti, U. (2011). The Evolution of Circumstellar Disks and their Dispersal: A Review. Recent Developments in Star Formation. Bangalore, India.
- Gorti, U. (2011, In Press). Disk Dispersal and Photoevaporation. BASI Conference Series.
- Hoehler, T.M. (2010). Compound extremes and energy limitation in aqueous environments on Mars: A case-study of methanogenesis in serpentinizing systems. 2010 Extremophiles Meeting. Azores, Portugal.
- Klingelhoefer, G., Morris, R.V., Blumers, M., Bernhardt, B. & Graff, T. (2011). The 2010 ILSO-ISRU field test at Mauna Kea, Hawai’i: Results from the miniaturized Moessbauer spectrometers MIMOS II and MIMOS IIA. Lunar Planet. Sci., XLII.
- McAdam, A.C., Ten Kate, I.L., Stern, J.C., Mahaffy, P.R., Blake, D.F., Morris, R.V., Steele, A., Amundson, H.E.F. & Team, A.2. (2010). Field characterization of the mineralogy and organic chemistry of carbonates from the 2010 Arctic Mars Analog Svalbard Expedition by evolved gas analysis. LPSC 42.
- McInerney, M.J., Hoehler, T.M. & Gunsalus, R.P. (2010). Introduction to hydrocarbon production: Bioenergetics. In: Timmis, K. (Eds.). Handbook of Hydrocarbon and Lipid Microbiology. Berlin: Springer-Verlag.
- Mercer, C.N. & Treiman, A.H. (2011). Energy produced from serpentinization of ultramafic rocks on terrestrial planets: Implications for sustaining microbial communities. AbGradConf.
- Morris, R.V., Ruff, S.W., Gellert, R., Ming, D.W., Arvidson, R.E., Clark, B.C., Golden, D.C., Siebach, K.L., Klingelhoefer, G., Schroeder, C., Fleischer, I., Yen, A.S. & Squyres, S.W. (2010). Discovery of carbonate-rich outcrops in the Gusev Crater Columbia Hills by the MER rover Spirit. AGU. San Francisco, CA.
- Parenteau, N. & Jahnke, L. (2011). Phototrophs and microfossils: What Yellowstone can teach us about the evolution of life on the early Earth and Mars. Montana State University Master in Science in Science Education Program. Bozeman, MT.
- Parenteau, N., Jahnke, L., Boomer, S., Cady, S. & Pierson, B. (2011). Do cyanobacteria use Fe(II) as an electron donor for photosynthesis? University of Hawai’i NAI Astrobiology Winter School, Hilo, HI.
- Parenteau, N., Jahnke, L., Cady, S. & Pierson, B. (2011). Cyanobacterial photoferrotrophy: Implications for the geochemical evolution of the early Earth and the rise of oxygen. Gordon Geobiology Conference. Ventura, CA.
- Parenteau, N., Jahnke, L., Siljeström, S. & Cady, S. (2011). Analysis of microbial mats: Lipid biomarker production and preservation in silica- and iron-depositing hot springs. Nordic – NASA Astrobiology Institute Research Project Meeting. Stockholm, Sweden.
- Parenteau, N., Valentine, P., Scapa, V., Jahnke, L., Boomer, S., Cady, S. & Pierson, B. (2010). Cyanobacterial photoferrotrophy: Implications for the geochemical evolution of the early Earth and the rise of oxygen. NAI Field Workshop: Anaerobic Phototrophic Ecosystems: Ancient and Modern. Fayetteville, NY.
- Perry, K.A., Bishop, J.L., Dyar, M.D., Blake, D.F., Peel, S. & Brown, A.J. (2011). Spectral analysis of nontronite-magnesite-olivine mixtures and implications for carbonates on Mars. LPSC 42.
- Quinn, J.E., Golden, D.C., Graff, T.G., Ming, D.W., Morris, R.V., Douglas, S., Kounaves, S.P., McKay, C.P., Tamparri, L.K., Smith, P.H., Zent, A.P. & Archer Jr., P.D. (2011). Chemistry and mineralogy of Antarctica Dry Valley soils: Implications for Mars. Lunar Planet. Sci., XLII.
- Sarrazin, P., Taylor, G.J., Blake, D., Vaniman, D. & Bish, D. (2011). XTRA: Extraterrestrial Regolith Analyzer. LPSC 42.
- Sutter, B., Ming, D.W., Boynton, W.V., Niles, P.B. & Morris, R.V. (2011). (Ca,Mg)-carbonate and Mg-carbonate at the Phoenix Landing Site: Evaluation of the Phoenix Lander’s Thermal Evolved Gas Analyzer (TEGA) using laboratory simulations. Lunar Planet. Sci., XLII.
- Tielens, A.G.G.M. & Allamandola, L.J. Cool Interstellar Physics and Chemistry. In: Khriachtchev, L. (Eds.). Physics and Chemistry at Low Temperature. Pan Stanford Publishing.
- Timmis, K. methanogenesis. In: Timmis, K. (Eds.). Handbook of Hydrocarbon and Lipid Microbiology. Berlin: Springer-Verlag.
- Wiens, R.C., Maurice, S., Bender, S., Barraclough, B.L., Cousin, A., Forni, O., Ollila, A., Newson, H., Vaniman, D., Clegg, S., Lasue, J.A., Blaney, D., DeFlores, L., Morris, R.V. & Team, t.C. (2011). Calibration of the MSL/ChemCam/LIBS remote sensing composition instrument. Lunar Planet. Sci., XLII.
- Wolfgang, A. & Laughlin, G. (2011, Submitted). Combining Kepler and HARPS Occurrence Rates to Infer the Period-Mass-Radius Distribution of Super-Earths/Sub-Neptunes. Astrophysical Journal.