2012 Annual Science Report
NASA Jet Propulsion Laboratory - Icy Worlds
Reporting | SEP 2011 – AUG 2012
Path to Flight
Project Summary
The (Field Instrumentation and) Path to Flight investigation’s purpose is to enable in-situ measurements of organics and biological material with field instrumentation that have high potential for future flight instrumentation. The preceding three Investigations (Habitability, Survivability and Detectability) provide a variety of measurable goals that are used to modify or “tune” instrumentation that can be placed in the field. In addition the members involved with Investigation provide new measurement capabilities that have been developed with the specific goal of life-detection and organic detection using both non-contact/non-destructive means and ingestion based methods. The developments under this investigation (Inv 4) incorporate state-of-the-art laboratory instruments and next generation in-situ instrumentation that have been developed under programs that include NASA as well as NSF and DOD. These include mass-spectrometers, gas analyzers, and fluorescence/Raman spectrometry instruments.
Project Progress
The (Field Instrumentation and) Path to Flight investigation’s purpose is to enable in-situ measurements of organics and biological material with field instrumentation that have high potential for future flight instrumentation. The preceding three Investigations provide a variety of measurable goals used to modify or “tune” instrumentation that can be placed in the field. In addition the members of this Investigation provide new measurement capabilities that have been developed with the specific goal of life-detection. The instrument arsenal goes beyond the commercially available instrumentation and brings next generation imaging spectrometers, chromatographic, and sample extraction devices.
The Path to Flight investigation has three (3) primary Objectives, where Objectives 2 and 3 were the primary focus of these years efforts – given the reduced budget. Below are the objectives and a list of accomplished tasks.
OBJECTIVE1: Chemistry at Hydrothermal Vents
1. Characterization of mineral species in Laboratory Hydrothermal Vent experiments (related to Inv 1 efforts)
OBJECTIVE3: Ground Truth and Observability
1. Preliminary finding from collaborative Development of Subsurface In-situ Microbial Detector (DEBI-T)
The following are some additional details associated to the accomplished tasks.
Chemistry at Hydrothermal Vents
In an effort to tie together the in-situ instrumentation being developed as future flight instruments, Raman spectroscopy was employed for analysis of hydrothermal vent chimneys being generated in Inv 1 by Ph.D candidate Lauren Spencer and Co-I M. Russell. The intent was to better understand the mineralogy of these chimneys and specifically the effect of temperature and its effect on mineralogical species. The primary components being observed were greigite, mackinawite, and Fe(III) containing mackinawite – all of which catalyze the synthesis of organic compounds. The results suggest an optimum formation of greigite occurs at temperatures similar to low-temperature hydrothermal vents – these results have been submitted as a paper and is currently being reviewed (Spencer et al. 2012, in submission).
In addition to the detection, characterization, and quantification of these mineral features, the results have fed back into instrument development efforts. During the acquisition of the Raman spectra, it was evident that increased power densities normally used for Raman analysis resulted in thermal alteration of greigite or mackinawite to magnetite. Magnetite was not formed natively in the chimney at lower growth temperatures. Thus, for in-situ Raman instruments, inadvertent thermal alteration needs to be taken into consideration. The effects of Raman analysis on both mineral and organic compounds with solutions for in-situ Raman instruments is the subject of a paper currently in preparation (Bhartia et al., 2012, in prep)
A) Simulated chimney formation under Hadean conditions B) ESEM image showing the interior and exterior the simulated chimney C) Raman spectroscopy of showing the prominent mineral phase in the simulated chimney as mackinawite; a catalyst for organic synthesis.
Ground Truth and Observability
This area represents the largest collective effort in Investigation 4; however, because of the significant expense involved with field instrumentation development, efforts for leveraged activities are essential.
During this year, our effort was placed on analyzing the data collected from a series of successful ocean/ocean subsurface deployments of the deep biosphere borehole instrument (DEBI-T) that was collaboratively built by Photon Systems, JPL, Lamont-Doherty Columbia Unviersity, and USC under funding from NSF, C-DEBI, Sloan foundation, and the NAI-Icy Worlds. The instrument is a novel deep ocean, deep UV native fluorescence instrument for subsurface in-situ detection of organics and microbes living on borehole walls and/or in the water column. The development of the instrument was spurred by results of label-free microbial detection that was demonstrated in part, in year 1 and 2 of the Icy Worlds NAI (Bhartia et al., 2010). In addition to supporting the Astrobiological goals of understanding the extent of life, the successful deployment of the instrument demonstrates a side-observing high pressure optical design that could be used to observe ice-boreholes in Greenland and Antarctica, analogs to future Europa missions, with the capability to withstand a tested pressures of greater than 10,000 psi.
Preliminary examination of the data show spectral features that are analogous to biological material at concentrations of that approach 1e5 cells/ml. These results are being compared to a database of standards that includes samples from bacterial cells, bacterial spores, endospores, and archea, and eukaryotes, as well and suspended colloidal particles and minerals to rule out effects from interferrants. The concentration of the biological material appears to increase with depth up to 450m and decreases after this suggesting that the observed material is native to the borehole. The results are under preparation for publication.
As a part of Investigation 4, we also continued to further develop the portable Mini 10 Mass Spectrometer (MS) system and reported its performance (Sokol et al., 2012) and demonstrated its feasibility in real field environment (Soparawalla et al., 2012). Field demonstration of the MS instrument has been done at the Mojave desert by an NAI summer graduate student Santosh Soparawalla of Purdue University. Air sampling was done by drawning air over sorbent tubes that were attached to a sample pump at a rate of 2 liters/min. Carbotrap C sorbent was used to look for aromatic hydrocarbons. During these tests the miniature MS system was able to detect the two most abundant components of diesel exhaust, naphthalene and acenaphthene, as well as other aromatic species likely to be a result of exhaust.
We also incorporated RCAL system into a JPL rover and demonstrated its performance at the JPL Mars Yard. RCAL had been mounted on the SRR-2K rover and all power and controls now come from the rover and successfully collected soil samples and analyze it. Earlier trials had identified shortcomings in some of the hardware and many actuators have now been replaced by encoded motors to give more reliable and precise control, coupled with appropriate changes to the operations software.
RCAL unit was mounted on the top of the JPL SRR-2K rover and all power and controls are supplied by the rover. Using the rover’s arm, soil samples were successfully collected at JPL’s Mars Yard (which mimic’s Martian surface rocks and soil) and delivered to RCAL system for analysis.
The recent discovery of water vapor plumes ejected from fissures near the south pole of Saturn’s satellite Enceladus compels us to point out the relevance of this icy satellite to the evolution of organics and possibly life in this unique physical and chemical environment. The Cassini data indicated that there is very likely a heated liquid subsurface ocean. Clearly, sufficient heat is present to generate the energetic flux of water vapor from the fissures and elevate the temperature of the surrounding region. Substantial subsurface spatial temperature gradients are expected. It is possible that weathering of rocks by liquid water occurs beneath the icy surface. Enceladus’ active hydrological cycle, where ice is heated and water vapor is expelled from the fissures), is a unique and promising new environment in which to trace organic chemical evolution and possibilities for life which inspired us for a new concept for a sample return mission called LIFE (Tsou et al., 2012).
Life Investigation For Enceladus (LIFE) presents a low-cost sample return mission to Enceladus, a body with high astrobiological potential. The LIFE mission concept is envisioned in two parts: first, to orbit Saturn (in order to achieve lower sampling speeds, approaching 2 km/s, and thus enable a softer sample collection impact than Stardust, and to make possible multiple flybys of Enceladus); second, to sample Enceladus’ plume, the E ring of Saturn, and the Titan upper atmosphere. With new findings from these samples, NASA could provide detailed chemical and isotopic and, potentially, biological compositional context of the plume. Since the duration of the Enceladus plume is unpredictable, it is imperative that these samples are captured at the earliest flight opportunity. The LIFE concept offers science returns comparable to those of a Flagship mission but at the measurably lower sample return costs of a Discovery-class mission.
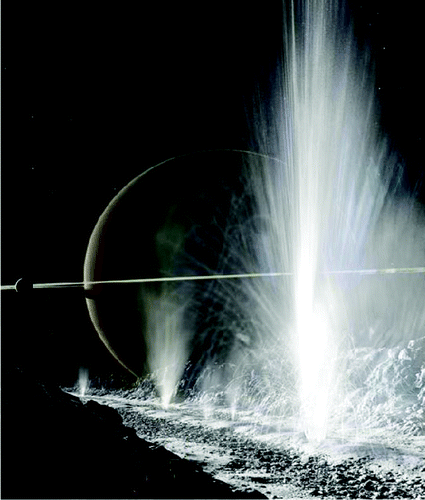
Artist’s conception of the Enceladus plume from the tiger stripes area with Saturn in view. In LIFE mission concept, the spacecraft would fly through the plume to collect samples of plume material for comprehensive analysis in terrestrial laboratories. Image credit: www.liebertonline.com/ast
References:
Bhartia, R., Salas, E. C.; Hug, W. F.; et al., Label-Free Bacterial Imaging with Deep-UV-Laser-Induced Native Fluorescence, (2010) APPLIED AND ENVIRONMENTAL MICROBIOLOGY, 76 Issue: 21 Pages: 7231-7237.
INVESTIGATION 4 – PUBLICATIONS:
Bhartia, Rohit, Everett C Salas, William F Hug, Ray D Reid, Arthur L Lane, Katrina J Edwards, and Kenneth H Nealson. “Label-Free Bacterial Imaging with Deep-UV-Laser-Induced Native Fluorescence.” (2012) Applied and Environmental Microbiology 76, no. 21: 7321–7237.
Bhartia, R., Hug, W.F., Fries, M., Reid, R., White, L., Salas E., “Sample Alteration and Solutions for Planetary Science” (2012) in prep for Astrobiology
White, L., Bhartia, R., Stucky G., Kanik, I., Russell, M., “Determining Catalytic Iron-sulfide Species in Ancient Alkaline Hydrothermal Vent Systems” submitted to Earth and Planetary Science (Sep 2012).
Sokol, E., Noll, R. J.; Cooks, R. G. et al., Miniature mass spectrometer equipped with electrospray and desorption electrospray ionization for direct analysis of organics from solids and solutions, (2011) INTERNATIONAL JOURNAL OF MASS SPECTROMETRY, 306, Issue: 2-3 Pages: 187-195 DOI: 10.1016/j.ijms.2010.10.019.
Tsou, P., Brownlee, D. E., McKay, C. P.; et al., LIFE: Life Investigation For Enceladus A Sample Return Mission Concept in Search for Evidence of Life , (2012) ASTROBIOLOGY, 12, Issue: 8 Pages: 730-742 DOI10.1089/ast.2011.0813
Presentations:
Soparawalla, S., Duncan, J.S., Ouyang, Z., Beegle, L. W., Fisher, A. M. , Kanik, I. and Cooks, R.G., In-situ Analysis of Organics, with a Portable Mass Spectrometer, ASCE, Engineering for Extreme Environments, Earth and space Conference (2012), Pasadena, California.
Publications
-
Bhartia, R., Salas, E. C., Hug, W. F., Reid, R. D., Lane, A. L., Edwards, K. J., & Nealson, K. H. (2010). Label-Free Bacterial Imaging with Deep-UV-Laser-Induced Native Fluorescence. Applied and Environmental Microbiology, 76(21), 7231–7237. doi:10.1128/aem.00943-10
-
Sokol, E., Noll, R. J., Cooks, R. G., Beegle, L. W., Kim, H. I., & Kanik, I. (2011). Miniature mass spectrometer equipped with electrospray and desorption electrospray ionization for direct analysis of organics from solids and solutions. International Journal of Mass Spectrometry, 306(2-3), 187–195. doi:10.1016/j.ijms.2010.10.019
-
Tsou, P., Brownlee, D. E., McKay, C. P., Anbar, A. D., Yano, H., Altwegg, K., … Kanik, I. (2012). LIFE: Life Investigation For Enceladus A Sample Return Mission Concept in Search for Evidence of Life. Astrobiology, 12(8), 730–742. doi:10.1089/ast.2011.0813
- Bhartia, R., Hug, W.F., Fries, M., Reid, R., White, L. & Salas, E. (In Preparation). Sample Alteration and Solutions for Planetary Science. Astrobiology.
- White, L., Bhartia, R., Stucky, G., Kanik, I. & Russell, M. (2012, Submitted). Determining Catalytic Iron-sulfide Species in Ancient Alkaline Hydrothermal Vent Systems. Earth and Planetary Science.
-
PROJECT INVESTIGATORS:
-
PROJECT MEMBERS:
Max Coleman
Co-Investigator
Kevin Hand
Co-Investigator
Michael Russell
Co-Investigator
Steven Vance
Co-Investigator
Robert Cooks
Collaborator
Ewa Sokol
Doctoral Student
Lauren Spencer-White
Graduate Student
-
RELATED OBJECTIVES:
Objective 1.1
Formation and evolution of habitable planets.
Objective 1.2
Indirect and direct astronomical observations of extrasolar habitable planets.
Objective 2.1
Mars exploration.
Objective 2.2
Outer Solar System exploration
Objective 3.1
Sources of prebiotic materials and catalysts
Objective 3.2
Origins and evolution of functional biomolecules
Objective 7.1
Biosignatures to be sought in Solar System materials
Objective 7.2
Biosignatures to be sought in nearby planetary systems