2011 Annual Science Report
University of Wisconsin
Reporting | SEP 2010 – AUG 2011
Project 5A: Production of Mixed Cation Carbonates in Abiologic and Biologic Systems
Project Summary
In the fourth year of our NAI project, a series of free drift experiments and one chemo-stat experiment were completed to determine the effect of temperature, chemical composition, pCO2, pH and precipitation rate on the incorporation of Mg in calcite in relatively dilute solutions. These experiments will permit geochemical relationships to be established between carbonate minerals and the solutions from which they form to gain a better understanding of the origin and occurrence of carbonates in ancient terrestrial and extraterrestrial environments.
Project Progress
The main focus of our research effort this year has been the continuation of experiments to grow Mg-bearing calcite inorganically from solution. Thirty-two free drift experiments and one chemo-stat experiment were performed to understand the effect of temperature, chemical composition, pCO2, pH, and precipitation rate on Mg-isotope fractionation in the calcite-aqueous Mg system.
Eighteen short term (2-7 days) free drift experiments were conducted with an initial solution composition of 50 mM MgCl2•6H2O, 15 mM CaCl2•2H2O, and 10 mM NaHCO3, over a range of values for pCO2 (0.3% to 3.0% CO2) and temperature (10° to 45°C). Fourteen long-term (38-58 days) free drift experiments were conducted with an initial solution composition of 30 to 50 mM Mg, 2.0 to 15 mM Ca, and 2.0 to 10 mM HCO3 at two values for pCO2 (0.038% and 3.0%) and a range of temperatures (4° to 35°); and a single chemostat experiment was conducted with an initial solution composition of 50 mM MgCl2•6H2O, 15 mM CaCl2•2H2O, and 10 mM NaHCO3, at a pCO2 of 0.3% CO2 and temperature of 22°C.
Fifty liter containers of relatively high concentration (e.g., 0.5 to 1.0 M) stock solution were mixed using the pure reagents; each container was then bubbled with pure CO2(g) until chemical equilibrium with the gas phase was attained (as determined by a constant solution pH). Specified aliquots of each stock solution were mixed in a reaction vessel with CO2(g)-equilibrated 18 M Ω cm de-ionized water to produce ~500 mL of master solution. The solution was re-equilibrated with a CO2/N2 gas mixture at a specified temperature until the solution restabilized at a higher pH value, resulting in the formation of a master solution that was slightly supersaturated with respect to Mg-calcite. The degree of supersaturation was controlled primarily by the pCO2 of the gas phase, resulting in a metastable solution that was incapable of spontaneous nucleation but sufficiently supersaturated to support the heterogenous growth of solid on a pre-existing carbonate template. At this juncture, calcite seed (~80 mg; Fig 1) was added to the master solution to initiate the formation of Mg-calcite overgrowths.
In the free-drift experiments, the solutions were continuously bubbled with a CO2/N2 gas mixture or the master solution was isolated from the environment by placing an airtight lid on the reaction vessel. In these experiments, the aqueous chemistry of the solution was permitted to drift toward chemical equilibrium over the course of an experiment. pH was continuously monitored over the course of each experiment. For the chemostat experiment, the aqueous chemistry of the solution was maintained in a metastable state of supersaturation by the addition of titrants that replenished the withdrawl of aqueous constituents consumed by the precipitation reaction. In this way, the aqueous chemistry, saturation state and precipitation state were fixed over the course of an experiment.
The duration of each experiment was chosen to ensure that sufficient solid was produced for the determination of the bulk and isotope composition Mg sequestered in the solid phase. At the conclusion of each experiment, an aliquot of solution was filtered and immediately analyzed for total alkalinity, and a second filtered sample was archived for the determination of aqueous Ca and Mg concentration, and Mg-isotope analysis by our NAI collaborators. The remaining solid was separated by filtration, rinsed briefly in DI water and dried for further physicochemical characterization. Final solid weight was used to estimate the percentage of overgrowth for comparison to changes in solution chemistry.
Aliquots of solid precipitate were examined by SEM to obtain high resolution secondary electron images of the overgrowths (Fig. 2). No textural features were observed in the final precipitate that permitted distinctions to be made between the seed material and the overgrowth. The most obvious textural difference between the seed and overgrowth was a significant roughening of the outer surface of the grains. Geochemical data (presented below), suggest the final solid consists of aggregates of particles that are cemented together by Mg-calcite overgrowths.
Several methods were used to determine the mole percentage of Mg in the overgrowth Aliquots of the final solid were characterized by x-ray diffraction, SEM, and electron microprobe using EDS and WDS analytical techniques. Two to four milligrams of the final solid was analyzed by x-ray diffraction after adding silicon as an internal standard. The presence of Mg-calcite was confirmed by a shoulder on the (104) reflection for calcite at ~29° 2θ (Fig. 3). The difference in 20 between the (104) peak for calcite and the (104) peak for Mg-calcite provides an estimate of the mol% MgCO3 in the overgrowth. Another two to four milligrams of solid were analyzed using an electron microprobe to quantify the Mg-content of the overgrowth by wave length dispersive spectroscopy (WDS) and energy dispersive spectroscopy (EDS). The solid was also digested and analyzed for Ca and Mg content using ICP-MS to confirm the mole percent MgCO3 in the overgrowth.
Most of the experiments (n=32) produced overgrowths that contained 0.1 to 12 mole% MgCO3 on pure calcite seed material. The spatial distribution of Mg in the overgrowths was determined from WDS-EDS scanning maps and quantitative analyses of sections of the final solid that were embedded in epoxy and polished (Fig. 4).
All of the free drift experiments conducted below 20°C at 3% pCO2 produced overgrowths containing < 1 mole% MgCO3. On the other hand, solutions of similar composition at a lower pCO2 (between 0.0385% and 1.65% pCO2) and temperature (4° and 15°C) produced overgrowth containing 2.5 to 9.1 mole% MgCO3. Experiments conducted at identical solution chemistries between 20° and 31°C formed overgrowths having 2.1 to 3.9 mole% MgCO3 at 3% pCO2. However, for these same solutions at a lower pCO2, the overgrowth contained 8.8 to 10.1 mole% MgCO3. At temperatures higher than 31°C, the overgrowth contained an elevated mole% MgCO3 compared to solids produced at a lower pCO2. At relatively low pCO2, the pH of the master solution was sufficiently high (>7) to promote the spontaneous nucleation of aragonite. Once formed, this phase played a dominant control on the aqueous chemistry of the solution because the relative precipitation rate for aragonite far exceeds that for Mg-calcite for the fluid compositions investigated in this study.
Given that solution chemistry changed throughout the course of the free drift experiments, it was likely that the overgrowths were chemically zoned. Therefore the geochemistry of the overgrowth was evaluated using a FESEM. For these analyses, the beam diameter was ~10 nm and the accelerating voltage was 10kV to minimize the interaction volume of the solid. Semi-quantitative results are presented below because standards were not analyzed, however, relative differences in concentration are meaningful by this method.
The results from FESEM analysis support the contention that the overgrowths were chemically zoned (Fig. 5). Line scans collected from all of the overgrowths showed that the grain cores contained pure calcite and rims were enriched in Mg, although the change in Mg concentration was lower than might be expected. This probably occurred because: 1) the aqueous Mg/Ca ratio did not change significantly, and 2) the mass concentration of aqueous constituents was relatively large compared to the mass of Mg and Ca sequestered in the solid phase over the course of an experiment.
Maintaining a constant chemical composition for the overgrowth is important for the characterization of precipitation kinetics and Mg-isotope fractionation for the calcite-aqueous Mg system. To avoid potential confounding factors, all future experiments will be performed using the chemo-stat technique, where solution chemistry is held constant throughout the entire course of an experiment. To date, one successful chemo-stat experiment has been performed at 22°C at 3% pCO2 and it produced an overgrowth of ~7 mole% MgCO3. Grains from this experiment and all future experiments will be analyzed by FESEM ensure that chemically homogeneous overgrowths are generated.
To summarize, the results our experiments reveal that temperature, solution composition and pCO2 all have an interrelated effect on the MgCO3 content and precipitation kinetics of Mg-calcite. Our experiments demonstrate that at relatively low temperature and high pCO2,the precipitation rate and MgCO3 content of Mg-calcite is low compared to experiments conducted at higher temperature and lower pCO2.
A matrix of chemostat experiments is presently being conducted so that the effects of solution composition (including Mg/Ca ratio), pH, pCO2, and precipitation rate can be evaluated independently for their effect on the incorporation of Mg into calcite. These data will guide efforts in the last year of our study to better understand how other constituents such as aqueous Fe and organic acids affect the geochemistry of carbonate minerals that form in low temperature environments.
Noteworthy results include:
1) Partial pressure of CO2 in solution is an effective means of controlling pH during an experimental run (for both free drift and chemo-stat experiment).
2) Use of large amount of seed crystals results in the generation of sufficient overgrowth for physicochemical characterization without having the solution chemistry change more than 10% over the course of a run for free drift experiment.
3) Temperature is an effective means of controlling precipitation rate and the percentage of overgrowth in an experimental run.
4) The use of calcite seed materials promotes the formation of Mg-calcite overgrowth without cross contamination from other phases such as aragonite or hydromagnesite.
5) XRD, ICP-MS and WDS/EDS can be used to characterize the physicochemical nature of the overgrowth.
6) FESEM studies suggest a micro scale inhomogeneity in the calcite overgrowth for free drift experiments. However, this problem can be overcome by using the chemo-stat experiment.
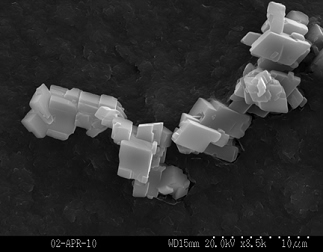
Figure 1. Secondary electron image (SEI) of calcite seed material. Laser particle diffraction analysis suggests the most common grain size of the seed was 20 microns in diameter, with a range of 4 to 50 microns. The SEI image shows these particles are actually aggregates of calcite rhombs as small as 2 microns.
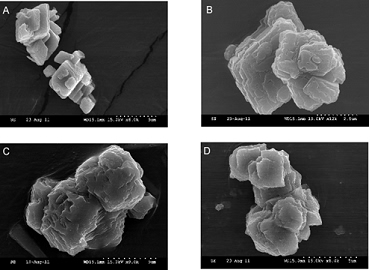
Figure 2. A. Calcite crystal with 0.8 mole% MgCO3 overgrowth. B. Calcite crystal with 2.7 mole% MgCO3 overgrowth. C. Calcite crystal with 7.8 mole% MgCO3 overgrowth. D. Calcite crystals with 10.1 mole% MgCO3 overgrowth.
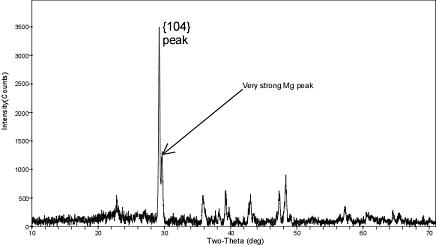
Figure 3. XRD diffractogram for solid from run 10-15-10-10C (pH = 7.48; 30ºC; 78% overgrowth; 10.09 mol% MgCO3). The pattern shows a distinct shoulder on the (104) calcite peak, which is diagnostic for the presence of a 10 mol% MgCO3 overgrowth.
Figure 4. Backscatter electron images and wavelength dispersive X-ray maps of the final solid (78% overgrowth) produced during run 10-15-10- at 30ºC (pH = 7.48; 10.09 ± 2.1 mol% MgCO3; n = 28 spot analyses).
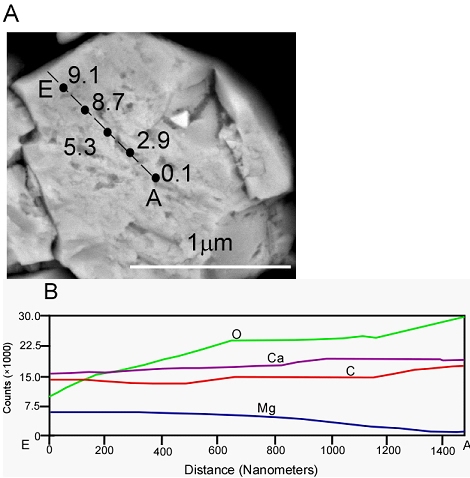
Figure 5. A. Secondary electron image showing quantitative EDS spot analyses performed on a calcite grain by FESEM (12-10-10-3:2-32). Mole% MgCO3 recorded next to spot. B. Line scan result of (A), showing elemental distribution across the overgrowth.
Publications
-
FERNÁNDEZ-REMOLAR, D. C., SÁNCHEZ-ROMÁN, M., Hill, A. C., GÓMEZ-ORTÍZ, D., Ballesteros, O. P., Romanek, C. S., & Amils, R. (2011). The environment of early Mars and the missing carbonates. Meteoritics & Planetary Science, 46(10), 1447–1469. doi:10.1111/j.1945-5100.2011.01238.x
-
Cloutis, E. A., Hudon, P., Romanek, C. S., Bishop, J. L., Reddy, V., Gaffey, M. J., & Hardersen, P. S. (2010). Spectral reflectance properties of ureilites. Meteoritics & Planetary Science, 45(10-11), 1668–1694. doi:10.1111/j.1945-5100.2010.01065.x
-
Jimenez-Lopez, C., Rodriguez-Navarro, C., Rodriguez-Navarro, A., Perez-Gonzalez, T., Bazylinski, D. A., Lauer, H. V., & Romanek, C. S. (2012). Signatures in magnetites formed by (Ca,Mg,Fe)CO3 thermal decomposition: Terrestrial and extraterrestrial implications. Geochimica et Cosmochimica Acta, 87, 69–80. doi:10.1016/j.gca.2012.03.028
-
Jimenez-Lopez, C., Romanek, C. S., & Bazylinski, D. A. (2010). Magnetite as a prokaryotic biomarker: A review. J. Geophys. Res., 115(G2), n/a–n/a. doi:10.1029/2009jg001152
-
Li, W., Chakraborty, S., Beard, B. L., Romanek, C. S., & Johnson, C. M. (2012). Magnesium isotope fractionation during precipitation of inorganic calcite under laboratory conditions. Earth and Planetary Science Letters, 333-334, 304–316. doi:10.1016/j.epsl.2012.04.010
-
Romanek, C. S., Morse, J. W., & Grossman, E. L. (2011). Aragonite Kinetics in Dilute Solutions. Aquat Geochem, 17(4-5), 339–356. doi:10.1007/s10498-011-9127-2
-
Sánchez-Román, M., McKenzie, J. A., De Luca Rebello Wagener, A., Romanek, C. S., Sánchez-Navas, A., & Vasconcelos, C. (2011). Experimentally determined biomediated Sr partition coefficient for dolomite: Significance and implication for natural dolomite. Geochimica et Cosmochimica Acta, 75(3), 887–904. doi:10.1016/j.gca.2010.11.015
-
Sánchez-Román, M., Romanek, C. S., Fernández-Remolar, D. C., Sánchez-Navas, A., McKenzie, J. A., Pibernat, R. A., & Vasconcelos, C. (2011). Aerobic biomineralization of Mg-rich carbonates: Implications for natural environments. Chemical Geology, 281(3-4), 143–150. doi:10.1016/j.chemgeo.2010.11.020
-
Yang, Y., Sahai, N., Romanek, C. S., & Chakraborty, S. (2012). A computational study of Mg2+ dehydration in aqueous solution in the presence of HS− and other monovalent anions – Insights to dolomite formation. Geochimica et Cosmochimica Acta, 88, 77–87. doi:10.1016/j.gca.2012.03.018
-
Zhao, W., Song, Z., Jiang, H., Li, W., Mou, X., Romanek, C. S., … Zhang, C. L. (2011). Ammonia-oxidizing Archaea in Kamchatka Hot Springs. Geomicrobiology Journal, 28(2), 149–159. doi:10.1080/01490451003753076
- Chakraborty, S., Romanek, C.S. & Moecher, D. (2011). Whole-rock geochemistry, mineralogy, petrography and thermometry of an L6 chondrite from Pembina country,. GSA. North Dakota.
-
PROJECT INVESTIGATORS:
-
PROJECT MEMBERS:
Max Coleman
Collaborator
Andrew Jacobson
Collaborator
Nadine Kabengi
Collaborator
Nita Sahai
Collaborator
Craig Schwandt
Collaborator
Suvankar Chakraborty
Postdoc
Nicholas Levitt
Doctoral Student
Didem Temel
Doctoral Student
Forrest Webb
Graduate Student
Olivia Woodruff
Graduate Student
-
RELATED OBJECTIVES:
Objective 4.1
Earth's early biosphere.
Objective 7.1
Biosignatures to be sought in Solar System materials