2011 Annual Science Report
Rensselaer Polytechnic Institute
Reporting | SEP 2010 – AUG 2011
Project 5: Vistas of Early Mars: In Preparation for Sample Return
Project Summary
To understand the history of life in the solar system requires knowledge of how hydrous minerals form on planetary surfaces, and the role these minerals play in the development of potential life forms. One hydrous mineral found on Earth and inferred from in situ measurements on Mars, is the mineral Jarosite, KFe3(SO4)2(OH)6. We are investigating whether radiometric ages, specifically 40Ar/39Ar ages on jarosite can be interpreted to accurately record climate change events on Mars. This project not only requires understanding the conditions required for jarosite formation and preservation on planetary surfaces, but also assessing under what conditions its “radiometric clock” can be reset (e.g., during changes in environmental conditions such as temperature). By studying jarosites formed by a variety of processes on Earth, we will be prepared to analyze and properly interpret ages measured from jarosite obtained from future Mars sample return missions.
Project Progress
The formation of supergene jarosite at planetary surfaces (i.e. Earth and Mars) requires the presence of water, however preservation of the mineral requires sustained aridity. As a potassium-bearing mineral, jarosite can be dated by the 40Ar/39Ar method. Therefore, jarosite 40Ar/39Ar ages can be used to date surface processes such as weathering and environmental transitions (i.e. aridification) on Earth and Mars. In preparation for Mars sample return missions and to better interpret jarosite ages from a thermochronological perspective, the diffusion kinetics of argon in jarosite were determined. Incremental fractional loss measurements indicate an activation energy (E) of 37.8 ± 1.5 kcal/mol and a log Do/a2 of 5.68 ± 0.63 s-1 corresponding to a closure temperature of 143 ± 28 °C, assuming a cooling rate 100°C/Ma (Figure 1).
Figure 1. Composite Arrhenius diagram from three size fractions of jarosite. Only points reflecting 39Ar released below 300°C are included. Above this temperature jarosite breaks down into hematite and yavapaiite.
Downward extrapolation of these parameters to Martian surface temperatures (≤ 22°C) predicts <1% fractional loss of Ar over 4.0 Ga. Forward modeling of 40Ar/39Ar age spectra using the least retentive E, Do/a2 pairs predict that if held at 22°C or less for 4.0 Ga, supergene jarosite would preserve original growth ages manifest as plateau ages consisting of >95% of the gas release (Figure 2).
Figure 2. Model jarosite 40Ar/39Ar age spectra based on diffusion kinetics summarized in Figure 1. A) Model results for jarosite held at 0°C for 4.0 Ga. Inset shows the full age spectra while the figure focuses on the initial 2.0% of the model gas release to highlight the partial loss profile. B) Model results for jarosite held at 22°C for 4.0 Ga. Inset shows the full age spectra while the figure focuses on the first 5.0% of the model gas release to highlight the partial loss profiles. Note both scenarios result in plateau ages within 1.0% of the 4.0 Ga crystallization age consisting of greater than 95% of the gas release.
Because of its susceptibility to mineralogical breakdown, 40Ar/39Ar ages on preserved Martian jarosite will reflect the time since water was present at a location that has since undergone aridification and remained hydrologically inactive and thermally quiescent.
As a proof of concept for applying jarosite 40Ar/39Ar chronometry to constrain the timing and rate of aridification for once-aqueous environments, a vertical profile of jarosite-bearing samples was collected from the top of the Paleocene Fort Union Formation in the Bighorn Basin of Wyoming. The Fort Union Formation exposed at the McDermotts Butte area (Figure 3) consists of interbedded fluvial sheet sandstones and floodplain mudstones (15, 16). Paleosols developed on the fine-grained floodplain deposits contain rhizoliths, relative abundances of goethite and hematite, and preserve jarosite indicating a general history of deposition, water saturation and soil formation, burial with sustained saturation, and finally excavation and drainage. This hydrologic sequence of saturation followed by drainage and sustained aridity (Figure 3) is analogous to that interpreted for the stratigraphy exposed at Burns Cliff at Meridiani Planum (Grotzinger et al., 2005).
Figure 3. Schematic representation of the Fort Union Formation in Wyoming (A) as a hydrologic analog for the Martian Burns Formation on Mars (B). T1-3 represent progressively younger positions of the water table with respect to the surface. T1 marks the time of deposition of the Fort Union Formation and the base eolian sand of the Burns Formation. Key times are T2 and T3 where, in both settings, the water table was at or above (ponds) the surface (T2) leaving the underlying sediments saturated and, following aridification, drained sediments sit above the water table (T3). Jarosite precipitation occurs between T2 and T3.
Jarosite precipitation during drainage of the Fort Union Formation is a time-transgressive process where the oldest jarosite (first-formed) should be at the top of the section and progressively younger jarosite should be present downsection. The ages measured from 5 jarosite samples collected along a 25 cm vertical profile are in agreement with this prediction. Jarosite ages systematically decrease downsection (Figure 4) indicating a jarosite precipitation front migrated downward through the
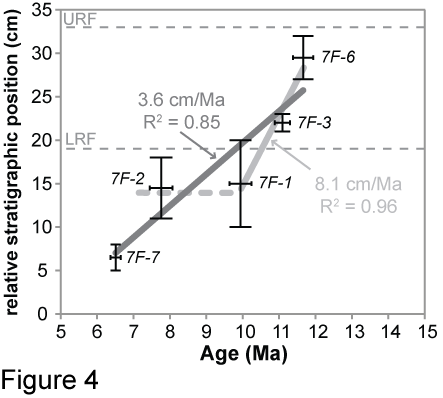
Figure 4. Plot showing correlation of stratigraphic position and age. Y-axis error bars reflect the size of the collected sample. X-axis error bars are 1σ uncertainty in age. Least-squares regression of all data (dark gray line) indicates an average drainage rate of 3.6 cm/Ma. Alternatively, the three highest samples define a drainage rate of 8.1 cm/Ma (light gray) followed by a possible ~2 Ma stagnation in water table position (dashed).
section at an average rate of 3.6 cm/Ma. The precipitation front likely reflects slow drainage through this portion of the sedimentary column. This result indicates that jarosite dissolution rates may be slower than those derived from laboratory experiments and that jarosite ages can record geologically slow environmental transitions over very small spatial distributions. Additionally, jarosite-bearing samples retrieved by future Mars sample return missions will afford determining when and how long water existed at the Mars surface.
Publications
-
Kula, J., & Baldwin, S. L. (2011). Jarosite, argon diffusion, and dating aqueous mineralization on Earth and Mars. Earth and Planetary Science Letters, 310(3-4), 314–318. doi:10.1016/j.epsl.2011.08.006
- Kula, J. & Baldwin, S.L. (2011). On the application of jarosite and hematite thermochronology to assess aqueous environments on Mars. Mineralogical Magazine (75): 1250
- Kula, J., Kraus, M.J., Brock-Hon, A.L. & Baldwin, S.L. (2011). Neogene drainage rates in the Bighorn Basin from jarosite 40Ar/39Ar chronometry, a hydrologic analog for Meridiani Planum, Mars. Geological Society of America Abstracts with Programs, 43: 675.
-
PROJECT INVESTIGATORS:
-
PROJECT MEMBERS:
John Delano
Co-Investigator
Bruce Watson
Co-Investigator
Joseph Kula
Postdoc
David Gombosi
Graduate Student
-
RELATED OBJECTIVES:
Objective 1.1
Formation and evolution of habitable planets.
Objective 2.1
Mars exploration.
Objective 7.1
Biosignatures to be sought in Solar System materials