2011 Annual Science Report
NASA Jet Propulsion Laboratory - Icy Worlds
Reporting | SEP 2010 – AUG 2011
Executive Summary
Our goal in the Astrobiology of the Icy Worlds Investigation is to advance our understanding of the role of ice in the broad context of astrobiology through a combined laboratory, numerical, analytical, and field investigations. Icy Worlds team will pursue this goal through four major investigations namely, the habitability, survivability, and detectability of life of icy worlds coupled with “Path to Flight” Technology demonstrations.
A search for life linked to the search for water should naturally “follow the ice”. Can life emerge and thrive in a cold, lightless world beneath hundreds of kilometers of ice? And if so, do the icy shells hold clues to life in the subsurface? These questions are the primary motivation of our science investigations which are as follows:
- Habitability of Icy Worlds investigates the habitability of liquid water environments in icy worlds, with a focus on what processes may give rise to life, what processes may sustain life, and what processes may deliver that life to the surface.
- Survivability of Icy Worlds investigates the survivability of biological compounds under simulated icy world surface conditions, and compare the degradation products to abiotically synthesized compounds resulting from the radiation chemistry on icy worlds.
- Detectability of Icy Worlds investigates the detectability of life and biological materials on the surface of icy worlds, with a focus on spectroscopic techniques, and on spectral bands that are not in some way connected to photosynthesis.
- Our technology investigation, a Path to Flight for astrobiology, utilizes instrumentation built with non-NAI funding to carry out the science investigations discussed above. The search for life requires instruments and techniques that can detect biosignatures from orbit and in-situ under harsh conditions. Advancing this capacity is the focus of our Technology Investigation.
The following sections highlight some of our accomplishments for the above investigations.
As far as the Habitability of Icy Worlds Progress is concerned, Co-I’s Drs. Russell and Kanik and team carried out a series of “origin of life” experiments using our hydrothermal vent reactor as a part of Investigation 1, Seafloor Processes (Fig. 1).
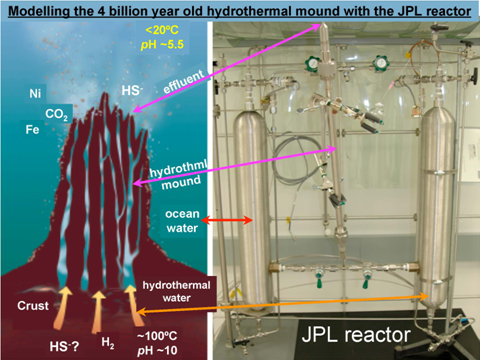
Figure 1. JPL Icy Worlds team researchers, Russell and Kanik, are experimentally testing the theory that life may have emerged ~4 billion years ago within porous and partly permeable submarine, moderate-temperature (≤100°C) alkaline hydrothermal springs on any wet, rocky planet or satellite in our solar system. Simulation experiments at JPL, modeling 4 billion-year-old hydrothermal mound, designed to test this theory, is shown.
The first experiment involved the interaction of carbonic and ammoniacal fluids with ultramafic silicate rock and sulfide such as would be found comprising the crust of our own and other wet (icy) rocky worlds. This experiment produced formate and amino acids, in particular alanine>>glycine>>serine and epsilon amino-n-caproic acid. Peptides and various amines are also recorded. The generation of peptides is particularly significant in that they feed into our hypothesis that sees the short peptides as the first emergent step to a functional organic world from inorganic beginnings (Milner-White and Russell, 2011). This paper sets the scene for further experimentation.
The results are consistent with our previously expressed view that the emergence of life on icy planets and moons was as inevitable as the onset of hydrothermal convection within their crusts—indeed, that a subaqueous convective system would have led directly to life’s emergence. The results also support similar arguments for the icy worlds elsewhere in the Universe.
Co-I Dr. Coleman and his collaborators continued their work in Investigation 1, Seafloor Processes by carrying out synthesis of abiotic organic matter through involvement in an exploration cruise to the area in which we discovered very deep high temperature hydrothermal vents in 2009. The results of this work will indicate the tectonic structure and context of the multiple hydrothermal vent fields. The Von Damm field was of particular interest, where we were able to observe a considerable and diverse fauna. The plume chemistry, sampled by CTD, suggests an ultramafic host rock but the ROV also observed, presumably basaltic, pillow lavas. A really diverse fauna could be observed including both more than one species of shrimp and hydrothermal tubeworms. This is a surprising discovery in two ways: shrimp and tubeworms together at a vent site and the presence of tubeworms in the Atlantic province.
Co-I Dr. Goodman and his students used the supercomputer system at Wheaton College to run three-dimensional plume dynamics models comparing the size, velocity, and temperature of plumes, incorporating equations of state for MgSO4 and water that take into account pressure-dependence of thermal expansion and heat capacity, properties held constant in conventional dynamics models for Earth’s oceans. Goodman also developed a single-column convection model, which will allow exploration the overall vertical structure of Europa’s ocean on a global scale.
Co-I’s Professor Brown and Dr. Vance conducted a focused set of sound velocity measurements in aqueous ammonia (a proxy for Titan’s ocean). Comparing new results with previous equations of state for solution density (Croft et al. 1988), they find that these previous results do not produce self-consistent forward-modeling results for sound velocity. Moreover, the new results reveal that sound velocities in aqueous ammonia show less concentration dependence than previously expected. Corresponding specific volumes (densities) are universally lower than previously predicted, strengthening arguments against extrusive ammonia-water cryo-volcanism on Titan.
Co-I Dr. Choukroun continued investigating clathrate formation, stability and dynamics and clathrate’s role in ice shell evolution in the astrobiological context. Experimental work examines the creation of clathrate hydrates. Computational modeling of ice shell convection tracks the shuttling of these and other biorelevant materials between oceans and surfaces, and also examines the possibility of “sub-glacial” lakes as briny oases within the ice. Recent experiments conducted in the H2O-CO2 system have shown a phase transition to a high-pressure phase of CO2 clathrate hydrates around 800 MPa. The structure of this phase exhibited strong similarities with the well-constrained high-pressure hexagonal phase of methane clathrate hydrates. Drs. Choukroun and Sotin continued to work on the transport of clathrate hydrates, salts, and biomolecules (i.e. determining the vertical velocity for realistic values of the viscosity) from the interior to the surfaces of icy satellites using simulation models the vertical velocity for realistic values of the viscosity.
As part of our Survivability of icy Worlds investigation, Co-Investigator Murthy Gudipati and his group continued studying the spectroscopy of ices and ices containing PAH impurities under UV and electron irradiation, aiming at understanding the chemistry of icy solar system surfaces such as Europa. In particular they have been successful in commissioning MALDI-TOF-MS experiment to investigate water-ices at any given temperature between 5 and 200 K. Their results clearly show that pure hydrocarbons, as soon as they are radiation processed and oxidized, can be strongly bound to the ice, making them less likely to exist as separate “organic phase”. An experiment conducted by the same investigators involving high energy electron bombardment of ices containing organic molecules produced a highly interesting result that electrons can damage organic molecules far deeper in ice than electron penetration depths predicted by models. These results are submitted for publication in ApJ.
As a part of Investigation 2, Co-Investigators (Drs. Johnson and Hodyss) have continued studying the photolysis of organic molecules in icy matrices relevant to solar system bodies. Their goal is to determine the precise chemical pathways that various molecules evolve in analog solar system ices as a function of temperature and wavelength. This involves photolysis of organics in ice, pure organics, matrix isolated organics, and organics under a layer of peroxide (to provide a source of hydroxyl radicals).
An extensive set of data have been collected on pholoysis of glycine, phenylalanine, oleic acid and SLPC. A paper has recently been submitted to the journal Astrobiology where a wavelength resolved study of UV photolysis of matrix-isolated glycine and phenylalanine is reported (Fig 2).
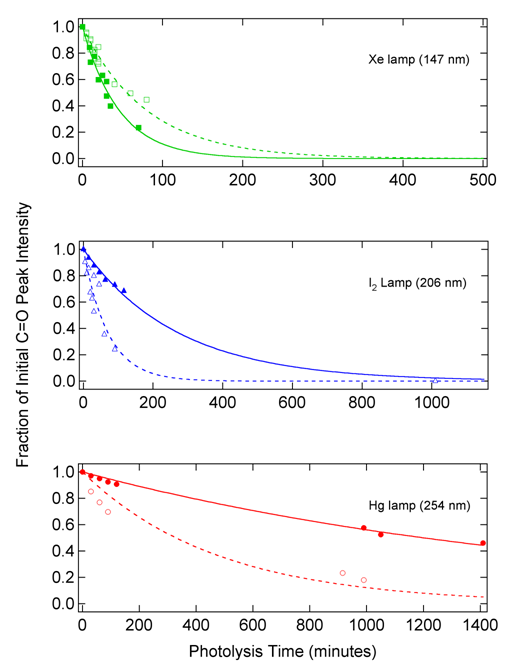
Figure 2. Photolytic decay curves for the carbonyl (C=O) stretch in glycine (solid symbols and solid lines) and phenylalanine (open symbols and dashed lines) as a function time exposed to (near) monochromatic UV radiation produced by the Xe (147nm), I2 (206nm), and Hg (254nm) lamps in the top, middle and bottom panels, respectively.
In all cases, decarboxylation is identified as the decomposition pathway. Further, we show that as a consequence of the significantly larger solar irradiance at longer wavelengths, it is the longer wavelength UV photons that will dominate amino acid destruction over more energetic, but less abundant, photons on icy bodies. Considering solar UV photolysis alone, we cannot reasonably expect to find amino acids in clean surface ices either remotely, or in situ at easily accessible depths. When the effects of other radiation sources such as the solar wind and magnetospheric particles (where applicable) are combined with UV photolysis and radical chemistry, it seems unlikely that organics can survive long enough on the surface of an icy planetary body to be detected without being frequently replenished from a shielded source such as an subsurface ocean.
Photochemical studies of thin cryogenic ice films composed of N2 and CH4 in ratios analogous to those on the surfaces of Neptune’s largest satellite, Triton, and on Pluto have been published in Icarus. Ultimately, this work suggests that C2-, CN, and CNN may be found in significant quantities on the surfaces of Triton and Pluto and that new observations of these objects in the appropriate wavelength regions are warranted.
A study of UV stimulated fluorescence and phosphorescence of benzene, toluene and the isomers of xylene in water ice has been published in the journal Astrobiology. The temperature dependence of the fluorescence and phosphorescence intensities was found to be independent of the thermal history and phase of the ice matrix in all cases. All phosphorescent emissions were found to decrease in intensity with increasing temperature. Similar behavior was observed for fluorescence in pure benzene, while the observed fluorescence intensity in water ices was independent of temperature. These results have implications for exploiting such phenomena for in situ detection of organics is solar system ices.
As a part of Investigation 2, Co-I Dalton has demonstrated that surface deposits on Europa contain both hydrated salts, frozen brines and sulfuric acid (Dalton et al., 2011a). (Fig 3).This confirms predictions of Carlson et al., (2005) that a radiolytic sulfur cycle is responsible for creation of sulfuric acid hydrate via chemical processing driven by magnetospheric charged particle bombardment. Dalton has now mapped the sulfuric acid distribution using Galileo Near-Infrared Mapping Spectrometer (NIMS) observations, and compared the sulfuric acid abundances with models of electron and ion energy deposited into the surface (Dalton et al., 2011b). The implications of this work suggest that while salts and brines in the surface deposits may be derived from an interior ocean, the sulfuric acid is “printed” over the surface irrespective of the underlying geologic unit. Dalton’s method provides a means of subtracting the sulfuric acid concentration and determining the relative concentrations of the remaining materials, which are linked to oceanic composition; in addition, the sulfuric acid concentration may provide a means of estimating exposure ages for young, disrupted areas. This will be useful in determining landing sites most likely to host viable biomolecules.
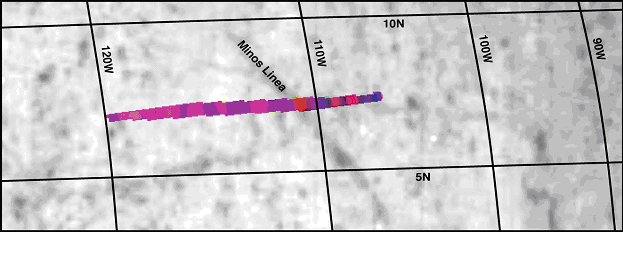
Figure 3. Context image taken with the Galileo Near-Infrared Mapping Spectrometer ‘s (NIMS) long spectrometer mode observation of Europa. The blue color identifies brighter, more icy terrain, while red is indicative of the presence of hydrated materials. Most pixels contain some of each, as shown by the purplish tones in the image.
Dalton’s analysis of the relationship between exogenically-produced sulfuric acid hydrate, and endogenically-derived hydrated magnesium and sodium sulfates and brines has already proven useful in selection of potential landing sites for a proposed Russian Europa lander mission (Ivanov et al., 2011; Dalton et al., 2011c).
Dalton has also demonstrated the presence of organic compounds on the Saturnian satellite Hyperion. Dark lag deposits in oddly shaped depressions exhibit spectral signatures indicative of both aromatic and aliphatic hydrocarbons, as seen by Cassini VIMS observations (Dalton et al., 2011d, submitted to Icarus). Additional, weaker signatures suggest the presence of nitrile (C-N bearing) compounds as well.
As a part of Investigation 2, Co‐investigator Dr. Cooper and group have been exploring the possibility of oxidant (O2 and H2O2) formation, for the interpretation of both surface and atmospheric composition of icy satellites, due to surface reactions of OH. The group has recently shown that methanol (CH3OH) was produced from the reaction of CH4 and O(1D) which is the dominant reaction channel for methanol formation although this reaction channel is mostly ignored in the literature in favor of the CH3 + OH reaction. They are continuing the matrix isolation work and will be extending to ice work in the near future to assess the relative importance of these channels in methanol formation.
In Investigation 2, Co-Investigator Dr. Gordon Love and his group at the University of California, Riverside, continued to investigate the production, preservation, and detection of chemotrophic lipid biological markers in Arctic thaw lakes as an analog for life detection under Icy Worlds conditions and generate historical, biomarker-based records of methane cycling and primary production in Arctic permafrost-bound lakes from sediment core analyses.
A fieldwork in October 2010 was conducted to collect sediment core samples which have all been freeze-dried and gently homogenized, completing the first step of the protocol developed for this project. Sedimentological descriptions of the cores have been compiled for communal use. Bulk inorganic geochemical analyses have been conducted on all October samples. Total lipid extracts have been obtained for all samples, and catalytic hydropyrolysis of whole sediment has been conducted on approximately half of the samples.
As a part of Investigation 2, Co-Is, Adrian Ponce and Kevin Hand carried out an investigation on the microbiology of Kilimanjaro glaciers. Microbial diversity data show that at the time of dust layer formation, the glacier surface hosted an active microbial, cold-water ecosystem. They found that a majority of bacterial clones, as determined by bacterial gene sequencing, are most closely related to those isolated from cold water environments. They also continued to investigate viability of spores and radiation resistant microbes in ices under radiation environment in astrobiological context (i.e Europa conditions). It should be noted hee that bacterial spores are the most resilient and long lived microbial forms, with report of longevity up to several hundred million years, making bacterial spores the most likely organisms to survive an interplanetary journey. Once embedded into Europan ice and subdued into its ocean by convection, the possibility of germination and outgrowth remains plausible. Strategies, including various detergents and drying conditions, in order to carry out the irradiation experiments have been developed and their suitability has been evaluated with electron microscopy. The experiments are expected to be carried out in early 2012.
In the area of Detection of Life in the Field, Co-I Kevin Hand and colleagues conducted two field campaigns in the Barrow, Alaska region 2011 to characterize and quantify methane release from the Alaskan North Slope region and to understand the origin and fate of the methane. This work focuses on a handful of thermokarst lakes near Barrow and extends to the roughly 13,000 lakes throughout the region, an unknown fraction of which are release methane to the atmosphere. From the experiments conducted to date we conclude that there is a great diversity of Archaea inhabiting these thermokarst lakes, which include, in part, a methanogenic community. These findings suggest that the archaeal community in lake sediments of the Northern Slope of Alaska may be more relevant to estimates of methane release from the Arctic than previously thought. This work will set the grounds to further the understanding of the effect of temperature increases on microbial activities that directly affect the greenhouse gas inventory, and it will expand the census of psychrophiles that thrive in permafrost environments and that could be used for testing under simulated Europa-like conditions.
As part of the Investigation, Hand and colleagues have identified and map lakes that may be releasing large quantities of methane using data from the Moderate Resolution Imaging Spectroradiometers (MODIS) onboard the Terra and Aqua satellites (Fig. 4). These satellites get near daily coverage of the Alaskan North Slope and one of the data products is a snow and ice coverage map for the region.
Future work will aim to ground truth these potentially methane releasing data points, helping to extend the methane mapping from the locale to the regional scale.
Figure 4. An example of a map generated using the 2004 MODIS data to locate possible methane seeping open water or non-snow covered lake ice regions for future investigations.
Field Instrument support of the Alaska campaigns is one of the major efforts for of Investigation 4 (Field Instrumentation and Path to Flight) activities. For this endeavor, a deep UV native fluorescence instrument (TUCBE – Targeted UV Chemical Biological Explorer – Photon Systems/JPL) whose development has been in part funded by DoD as well as NASA Planetary Protection Research, will be deployed in the coming fiscal year. Under the NAI hardening of the scanning pan/tilt stage has been completed. For a local test prior to deployment in Alaska, we successfully deployed the instrument in the Mojave and demonstrated capability to operate in a bright, high UV, outdoor environment, as well as detect organics on natural surfaces. These results were confirmed from air measurements that were conducted by a portable miniature mass spectrometer that was also tested in the field (Mojave Desert). A Carbotrap C sorbent was used to capture for aromatic hydrocarbons which subsequently introduced into the MS. During these tests the miniature MS system was able to detect the two most abundant components of diesel exhaust, naphthalene and acenaphthene, as well as other aromatic species likely to be a result of exhaust.
In addition to the Alaska field endeavor, a new deep ocean, deep UV native fluorescence instrument for subsurface in-situ detection of organics and microbes living on borehole walls (DEBI-T) was designed, developed, tested and was successfully deployed in North Pond located near the Mid-Atlantic Ridge. The successful deployment of the instrument demonstrates a side-observing high pressure optical design that could be used to observe ice-boreholes in Greenland and Antarctica, analogs to future Europa missions, with the capability to withstand a tested pressures of greater than 10,000 psi.
As a part of Investigation 4, Path to Flight, PI Kanik and colleagues, designed a low-cost flyby concept for a sample return mission called LIFE to Enceladus, a body with high astrobiological potential. A mission opportunity such as LIFE is extremely rare: it would offer science returns comparable to that of Flagship mission but at a much lower flyby sample return cost as a Discovery Mission. The LIFE mission concept is envisioned to orbit Saturn (both to achieve lower sampling speeds approaching 2 km/s which would enable more gentle sample collection than Stardust and to make possible multiple flybys of Enceladus), to sample Enceladus’ plume, the E ring of Saturn and the Titan upper atmosphere (Fig 5). LIFE could take advantage of a Jupiter gravity assist if it is launched before 2019 to reduce mission life times and also launch vehicle costs.
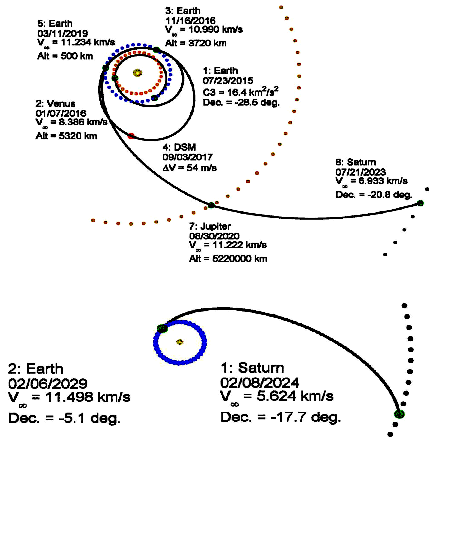
Figure 5. Typical LIFE Trajectory. The outbound trajectory departs Earth for a Venus, Earth and Jupiter gravity assists respectively then a Saturn Orbit Insertion. After 8 months Saturn tour, deorbits Saturn for 5 year Earth direct return.
References:
Milner-White EJ, Russell MJ 2011, A peptide era heralding the emergence of life. Genes 2, 671-688
Publications
-
Barge, L. M., Doloboff, I. J., White, L. M., Stucky, G. D., Russell, M. J., & Kanik, I. (2012). Characterization of Iron–Phosphate–Silicate Chemical Garden Structures. Langmuir, 28(8), 3714–3721. doi:10.1021/la203727g
-
Bhartia, R., Salas, E. C., Hug, W. F., Reid, R. D., Lane, A. L., Edwards, K. J., & Nealson, K. H. (2010). Label-Free Bacterial Imaging with Deep-UV-Laser-Induced Native Fluorescence. Applied and Environmental Microbiology, 76(21), 7231–7237. doi:10.1128/aem.00943-10
-
Choukroun, M., & Grasset, O. (2010). Thermodynamic data and modeling of the water and ammonia-water phase diagrams up to 2.2 GPa for planetary geophysics. J. Chem. Phys., 133(14), 144502. doi:10.1063/1.3487520
-
Cooper, P. D. (2012). Oxygen in the Outer Solar System. Journal of Chemical Education, 89(2), 181–182. doi:10.1021/ed200013q
-
Dalton, J. B., Cruikshank, D. P., Stephan, K., McCord, T. B., Coustenis, A., Carlson, R. W., & Coradini, A. (2010). Chemical Composition of Icy Satellite Surfaces. Space Sci Rev, 153(1-4), 113–154. doi:10.1007/s11214-010-9665-8
-
Dalton, J. B., Shirley, J. H., & Kamp, L. W. (2012). Europa’s icy bright plains and dark linea: Exogenic and endogenic contributions to composition and surface properties. Journal of Geophysical Research: Planets, 117(E3), n/a–n/a. doi:10.1029/2011je003909
-
Davies, A. G., Sotin, C., Matson, D. L., Castillo-Rogez, J., Johnson, T. V., Choukroun, M., & Baines, K. H. (2010). Atmospheric control of the cooling rate of impact melts and cryolavas on Titan’s surface. Icarus, 208(2), 887–895. doi:10.1016/j.icarus.2010.02.025
-
Fortes, A. D., & Choukroun, M. (2010). Phase Behaviour of Ices and Hydrates. Space Sci Rev, 153(1-4), 185–218. doi:10.1007/s11214-010-9633-3
-
Garozzo, M., Fulvio, D., Kanuchova, Z., Palumbo, M. E., & Strazzulla, G. (2009). The fate of S-bearing species after ion irradiation of interstellar icy grain mantles. A&A, 509, A67. doi:10.1051/0004-6361/200913040
-
Hand, K. P., & Carlson, R. W. (2011). H2O2 production by high-energy electrons on icy satellites as a function of surface temperature and electron flux. Icarus, 215(1), 226–233. doi:10.1016/j.icarus.2011.06.031
-
Hand, K. P., & Carlson, R. W. (2012). Laboratory spectroscopic analyses of electron irradiated alkanes and alkenes in solar system ices. Journal of Geophysical Research: Planets, 117(E3), n/a–n/a. doi:10.1029/2011je003888
-
Hand, K. P., Khurana, K. K., & Chyba, C. F. (2011). Joule heating of the south polar terrain on Enceladus. Journal of Geophysical Research, 116(E4), None. doi:10.1029/2010je003776
-
Hodyss, R., Howard, H. R., Johnson, P. V., Goguen, J. D., & Kanik, I. (2011). Formation of radical species in photolyzed CH4:N2 ices. Icarus, 214(2), 748–753. doi:10.1016/j.icarus.2011.05.023
-
Ivanov, M. A., Prockter, L. M., & Dalton, B. (2011). Landforms of Europa and selection of landing sites. Advances in Space Research, 48(4), 661–677. doi:10.1016/j.asr.2011.05.016
-
Johnson, P. V., Hodyss, R., Bolser, D. K., Bhartia, R., Lane, A. L., & Kanik, I. (2011). Ultraviolet-Stimulated Fluorescence and Phosphorescence of Aromatic Hydrocarbons in Water Ice. Astrobiology, 11(2), 151–156. doi:10.1089/ast.2010.0568
-
Johnson, P. V., Hodyss, R., Chernow, V. F., Lipscomb, D. M., & Goguen, J. D. (2012). Ultraviolet photolysis of amino acids on the surface of icy Solar System bodies. Icarus, 221(2), 800–805. doi:10.1016/j.icarus.2012.09.005
-
Korablev, O., Gerasimov, M., Brad Dalton, J., Hand, K., Lebreton, J-P., & Webster, C. (2011). Methods and measurements to assess physical and geochemical conditions at the surface of Europa. Advances in Space Research, 48(4), 702–717. doi:10.1016/j.asr.2010.12.010
-
Lorenz, R., & Sotin, C. (2010). The Moon That Would Be a Planet. Scientific American, 302(3), 36–43. doi:10.1038/scientificamerican0310-36
-
McGlynn, S. E., Kanik, I., & Russell, M. J. (2012). Peptide and RNA contributions to iron-sulphur chemical gardens as life’s first inorganic compartments, catalysts, capacitors and condensers. Philosophical Transactions of the Royal Society A: Mathematical, Physical and Engineering Sciences, 370(1969), 3007–3022. doi:10.1098/rsta.2011.0211
-
Mielke, R. E., Robinson, K. J., White, L. M., McGlynn, S. E., McEachern, K., Bhartia, R., … Russell, M. J. (2011). Iron-Sulfide-Bearing Chimneys as Potential Catalytic Energy Traps at Life’s Emergence. Astrobiology, 11(10), 933–950. doi:10.1089/ast.2011.0667
-
Modica, P., & Palumbo, M. E. (2010). Formation of methyl formate after cosmic ion irradiation of icy grain mantles. A&A, 519, A22. doi:10.1051/0004-6361/201014101
-
Nitschke, W., & Russell, M. J. (2011). Redox bifurcations: Mechanisms and importance to life now, and at its origin. BioEssays, 34(2), 106–109. doi:10.1002/bies.201100134
-
Orzechowska, G. E., Kidd, R. D., Foing, B. H., Kanik, I., Stoker, C., & Ehrenfreund, P. (2011). Analysis of Mars analogue soil samples using solid-phase microextraction, organic solvent extraction and gas chromatography/mass spectrometry. International Journal of Astrobiology, 10(03), 209–219. doi:10.1017/s1473550410000443
-
Palumbo, M. E., Baratta, G. A., Leto, G., & Strazzulla, G. (2010). H bonds in astrophysical ices. Journal of Molecular Structure, 972(1-3), 64–67. doi:10.1016/j.molstruc.2009.12.017
-
Pearce, M. P., Bussemaker, M. J., Cooper, P. D., Lapere, K. M., Wild, D. A., & McKinley, A. J. (2012). Formation of methanol from methane and water in an electrical discharge. Physical Chemistry Chemical Physics, 14(10), 3444. doi:10.1039/c2cp22135g
-
Robuchon, G., Choblet, G., Tobie, G., Čadek, O., Sotin, C., & Grasset, O. (2010). Coupling of thermal evolution and despinning of early Iapetus. Icarus, 207(2), 959–971. doi:10.1016/j.icarus.2009.12.002
-
Shirley, J. H., Dalton, J. B., Prockter, L. M., & Kamp, L. W. (2010). Europa’s ridged plains and smooth low albedo plains: Distinctive compositions and compositional gradients at the leading side–trailing side boundary. Icarus, 210(1), 358–384. doi:10.1016/j.icarus.2010.06.018
-
Simoncini, E., Russell, M. J., & Kleidon, A. (2011). Modeling Free Energy Availability from Hadean Hydrothermal Systems to the First Metabolism. Orig Life Evol Biosph, 41(6), 529–532. doi:10.1007/s11084-011-9251-4
-
Sohl, F., Choukroun, M., Kargel, J., Kimura, J., Pappalardo, R., Vance, S., & Zolotov, M. (2010). Subsurface Water Oceans on Icy Satellites: Chemical Composition and Exchange Processes. Space Sci Rev, 153(1-4), 485–510. doi:10.1007/s11214-010-9646-y
-
Sokol, E., Noll, R. J., Cooks, R. G., Beegle, L. W., Kim, H. I., & Kanik, I. (2011). Miniature mass spectrometer equipped with electrospray and desorption electrospray ionization for direct analysis of organics from solids and solutions. International Journal of Mass Spectrometry, 306(2-3), 187–195. doi:10.1016/j.ijms.2010.10.019
-
Tsou, P., Brownlee, D. E., McKay, C. P., Anbar, A. D., Yano, H., Altwegg, K., … Kanik, I. (2012). LIFE: Life Investigation For Enceladus A Sample Return Mission Concept in Search for Evidence of Life. Astrobiology, 12(8), 730–742. doi:10.1089/ast.2011.0813
-
Wolfe-Simon, F., Blum, J. S., Kulp, T. R., Gordon, G. W., Hoeft, S. E., Pett-Ridge, J., … Oremland, R. S. (2010). A Bacterium That Can Grow by Using Arsenic Instead of Phosphorus. Science, 332(6034), 1163–1166. doi:10.1126/science.1197258
- (2011). Astrobiology of Icy Worlds: Photochemistry of Planetary Ices. Physics Colloquium (MH 606). California State University, Fullerton, California.
- Adams, H.E. & Priscu, J.C. (2011). Bacterial activity in methane-rich, icy habitats. American Society for Limnology and Oceanography, Aquatic Sciences Meeting. San Juan, Puerto Rico.
- Adams, H.E. & Priscu, J.C. (2011, In Preparation). Methane oxidation in icy habitats.
- Adams, H.E., Hand, K.P., Murray, A.E., Lyons, B. & Priscu, J.C. (In Preparation). Distribution of bacterial communities, activity, and carbon sources in Alaskan coastal plain lakes.
- Beegle, L.W., Kim, H.I., Kanik, I. & Ryu, E.K. (2011). NASA Tech Briefs “Improved Ambient Pressure Pyroelectric Ion Source”.
- Choukroun, M., Kieffer, S.W. & Lu X., T.G. (In Press). Clathrate hydrates: Implications for Exchange Processes in the outer Solar System [Book Chapter]. Solar System Ices.
- Cooper, P.D., Do, N., Strube, A. & Ammann, L.M. (2010). Oxidant Loading of Icy Satellite Surfaces by Re-deposition and Subsequent Surface Chemistry. DPS. Pasadena CA.
- Dalton, I.J.B., Shirley, J.H., Cassidy, T., Paranicas, C.J. & Kamp, L.W. (2011b). Surface effects of endogenic and exogenic processes on Europa. EPSC-DPS Joint Meeting, 6(649).
- Dalton, J.B., Prockter, L.M. & Ivanov, M.A. (2011c). Surface materials on Europa: Considerations for Landing Site Selection. Proc. 2nd Moscow Solar System Symposium (2M-S3). Moscow.
- Dalton, J.B., Shirley, J.H. & Prockter, L.M. (2010). Surface geology of Europa: A window to subsurface composition and habitability. Geophysical Research Abstracts, 12.
- Do, N., Strube, A., Ammann, L.M. & Cooper, P.D. (2011, Submitted). A Laboratory Investigation of the Chemistry of Resurfacing on Icy Bodies. Ap. J.
- Duong, T.A. & Kanik, I. (2011). DIFFERENTIAL MOBILITY SPECTROMETER WITH SPATIAL ION DETECTOR AND METHODS RELATED THERETO [Patent]. DIFFERENTIAL MOBILITY SPECTROMETER WITH SPATIAL ION DETECTOR AND METHODS RELATED THERETO.
- Eiceman, G.A., Coy, S., Mielke, R. & Kanik, I. (2011, In Preparation). Detection of Organic Compounds with a Field Portable Membrane Introduction Differential Mobility Spectrometer System.
- Gleeson, D.F., Amashukeli, X. & Pappalardo, R.T. An investigation into biologically mediated sulfur cycling under simulated Europa ocean conditions.
- Hand, K.P. & Painter, T.H. (2010). Fall AGU Mtg.
- Hand, K.P. (2011). On the Coming Age of Ocean Exploration. In: Brockman, M. (Eds.). Future Science.
- Hand, K.P. Outer planet satellites as potential crucibles for life: extraterrestrial and terrestrial. UN meeting.
- Hodyss, R. (2011). New Horizon Workshop on Icy Surface Processes. Flagstaff Arizona.
- Hodyss, R., Howard, H.R., Johnson, P.V., Goguen, J.D. & Kanik, I. (To Be Submitted). Atomic Carbon Chemistry in Photolyzed Triton-Like Ices. Icarus.
- Hodyss, R., Kim, H.I., Bolser, D.K., Johnson, P.V., Goguen, J.D. & Kanik, I. (To Be Sbmitted). Ultraviolet Photolysis of Lipids. Icarus.
- Hussmann, H., Choblet, G., Lainey, V., Matson, D., Sotin, C., Tobie & Van Hoolst, T. (2010). Internal Processes: Energy Sources and Heat Transport in Icy Satellites. In: Grasset et al. (Eds.). Internal Processes: Energy Sources and Heat Transport in Icy Satellites.
- Johnson, P. (2010). Atomic Carbon Chemistry in Photolyzed Triton-like Ices. 2010 Fall AGU Meeting. San Francisco, CA.
- Johnson, P.V., Hodyss, R., Howard, H.R., Goguen, J.D. & Kanik, I. (2010). Atomic Carbon Chemistry in Photolyzed Triton-like Ices. 42nd annual meeting of the Division for Planetary Sciences of the American Astronomical Society. Pasadena, CA.
- Milner-White, E.J. & Russell, M.J. (2011). , A peptide era heralding the emergence of life. Genes, 2: 671-688.
- Pearce, M., McKinley, A.J. & Cooper, P.D. (In Preparation). Formation of Methanol in Argon Matrices. Chem. Phys. Phys. Chem.
- Russell, M.J. (2011). Origins, Abiogenesis and the search for Life. Cambridge: Cosmology Science.
- Russell, M.J. (2011). The origin of life. Encyclopaedia of Geobiology. Netherlands: Reitner, J and Thiel, V. Singer.
- Schoepp-Cothenet, B., v.L., R., P., P., M., A., R-W. & Russell, M.J. (2011). A peptide era heralding the emergence of life. Genes, 2: 671-688.
- Schubert, G., Hussmann, H., Lainey, V., Matson, D., McKinnon, W., Sohl, F., Sotin, C., Tobie, G., Turrini, D. & Van Hoolst, T. Evolution of the Moons; Europlanet. Icy Satellites.
- Soparawalla, S., Duncan, J.S., Ouyang, Z., Beegle, L.W., Fisher, A.M., Kanik, I. & Cooks, G.R. (2012). In Situ Analysis of Organics with a Portable Mass Spectrometer. Engineering in Extreme Environment, Earth and Space 2012 Conference. Pasadena CA.
- Soparawalla, S., Duncan, J.S., Ouyang, Z., Beegle, L.W., Fisher, A.M., Kanik, I. & Cooks, G.R. In Situ Analysis of Organics with a Portable Mass Spectrometer. To be presented at ASCE, Engineering in Extreme Environment.
- StrazStrazzulla, G. Ion Bombardment of astrophysical iceszulla, G. Ion Bombardment of astrophysical ices. 24th International Conference on atomic collision in solids. Cracow, Poland.
- Tsou, P., Brownlee, D.E., McKay, C.P., Yano, H., Strange, N., Beegle, L.W., Dissley, R. & Kanik, I. (2011). LIFE: Life Investigation For Enceladus, A Sample Return Mission Concept in Search for Evidence of Life. AGU Fall meeting. San Francisco, CA.