2011 Annual Science Report
Arizona State University
Reporting | SEP 2010 – AUG 2011
Astrophysical Controls on the Elements of Life, Task 6: Determine Which Elemental or Isotopic Ratios Correlate With Key Elements
Project Summary
In this task we explore how key elements and radioactive isotopes are created by nucleosynthesis during the explosions of massive stars. We also study the formation and composition of structures in supernova explosions that may be relevant to delivery of bioessential elements to forming solar systems. In particular, we have investigated how the bioessential elements Ca and Fe are produced during supernovae. We have discovered that they are produced by 6 distinct nucleosynthetic pathways, and that their relative abundances can be probed by observations of the gamma-ray radiation from the radioactive decay of the isotopes 44Ti and 56Ni into 44Ca and 56Fe. We also have investigated the co-production of O isotopic anomalies with the short-lived radionuclide 26Al. We find that delivery of 26Al to the early solar system would not necessarily have altered significantly its O isotopic composition.
Project Progress
Injection of 26Al from a nearby supernova into our forming solar system is an attractive explanation for the origin of this short-lived radionuclide (SLR). Previous work had suggested that supernova delivery of 26Al into a protoplanetary disks would dramatically alter the oxygen isotopic composition of solar system bodies in measureable and unseen ways. Comparing nucleosynthesis in clumps of matter from supernova simulations to meteoritic data and the solar composition determined by the Genesis mission, Patrick Young (ASU), Carola Ellinger (ASU/UT Arlington), and Steve Desch (ASU) demonstrate that supernova injection can be consistent with solar system oxygen isotopic abundances. This work was published in December 2010.
Abundances of material injected into disks or molecular clouds depend on the methods of delivery. Simulations Steve Desch, Frank Timmes, Evan Scannapieco and Liubin Pan (ASU) indicate that dense clumps of material and dust formed in them are the most effective delivery methods. Patrick Young (ASU), Carola Ellinger (ASU/UT Arlington), Chris Fryer (Los Alamos), and Gabe Rockefeller (Los Alamos) performed a large suite of supernova simulations to determine how structures form in explosions and what mix of elements they contain. We have developed a method to quantify the size spectrum of clumps that can be compared to observations.
Detecting γ-rays from the decay of radioactive isotopes in supernova remnants or accumulated in interstellar medium provides a direct calibration of nucleosynthesis in core-collapse events. For example, decay of 44Ti and 56Ni has observational consequences for light curves of supernovae, isotopic patterns in primitive meteorites and presolar grains, anomalies in deep-sea crusts, and solar abundances of calcium and iron. Georgios Magkotsios (Notre Dame), F.X. Timmes (ASU), Aimee Hungerford (Los Alamos), Chris Fryer (Los Alamos), Patrick Young (ASU) and Michael Wiescher (Notre Dame) compare the yields of 44Ti and 56Ni produced from post-processing thermodynamic trajectories of materials in the explosion from three core-collapse models with exponential and power-law trajectories. We found six nucleosynthesis regions in the peak temperature and density plane, all of which are reached by these models.

Figure 1: Simulation of an asymmetric supernova explosion approximately six months after the explosion. Color gradients indicate density. Clumps in the outer regions are produced by Rayleigh Taylor instabilities, while interior structures are bubble walls caused by heating from radioactive decay of 56Ni.
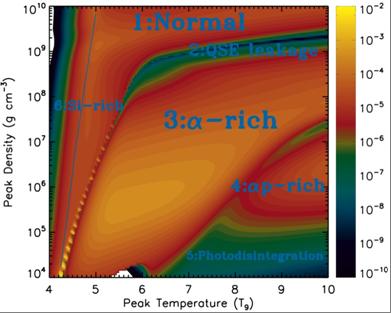
Figure 2: Final mass fraction of 44Ti in the peak temperature-density plane for an thermodynamic profile for symmetric matter. Six distinct regions of 44Ti synthesis are labeled. Region 1: normal freeze-out from Nuclear Statistical Equilibrium, abundance largely determined from Q values. Region 2: Chasm region, passage from 1 Quasi-static Equilibrium cluster to 2 Quasi-static Equilibrium clusters. Region 3: α-rich freeze-out. Region 4: αp- rich freeze-out. Region 5:Photodisintegration regime, neutrons, protons, and α particles dominate. Region 6: Incomplete silicon burning.
Publications
-
Ellinger, C. I., Young, P. A., & Desch, S. J. (2010). COLLATERAL EFFECTS ON SOLAR NEBULA OXYGEN ISOTOPES DUE TO INJECTION OF 26 Al BY A NEARBY SUPERNOVA. The Astrophysical Journal, 725(2), 1495–1506. doi:10.1088/0004-637x/725/2/1495
-
Magkotsios, G., Timmes, F. X., Hungerford, A. L., Fryer, C. L., Young, P. A., & Wiescher, M. (2010). TRENDS IN 44 Ti AND 56 Ni FROM CORE-COLLAPSE SUPERNOVAE. The Astrophysical Journal Supplement Series, 191(1), 66–95. doi:10.1088/0067-0049/191/1/66
-
PROJECT INVESTIGATORS:
-
PROJECT MEMBERS:
Chris Fryer
Collaborator
Gabriel Rockefeller
Collaborator
Carola Ellinger
Graduate Student
Aimee Hungerford
Unspecified Role
Georgios Magkotsios
Unspecified Role
Michael Wiescher
Unspecified Role
-
RELATED OBJECTIVES:
Objective 1.1
Formation and evolution of habitable planets.
Objective 3.1
Sources of prebiotic materials and catalysts