2009 Annual Science Report
NASA Jet Propulsion Laboratory - Icy Worlds
Reporting | JUL 2008 – AUG 2009
Astrobiology of Icy Worlds
Project Summary
Icy worlds such as Titan, Europa, Enceladus, and others may harbor the greatest volume of habitable space in the Solar System. For at least five of these worlds, considerable evidence exists to support the conclusion that oceans or seas may lie beneath the icy surfaces. The total liquid water reservoir within these worlds may be some 30 to 40 times the volume of liquid water on Earth. This vast quantity of liquid water raises two questions: Can life emerge and thrive in such cold, lightless oceans beneath many kilometers of ice? And if so, do the icy shells hold clues to life in the subsurface? We will address these questions through four major investigations namely, the habitability, survivability, and detectability of life of icy worlds coupled with “Path to Flight” Technology demonstration. We will also use a wealth of existing age-appropriate educational resources to convey concepts of astrobiology, spectroscopy, and remote sensing; develop standards-based, hands-on activities to extend the application of these resources to the search for life on icy worlds.
Project Progress
Our Goal is to advance our understanding of the role of ice in the broad context of astrobiology through collaborative investigations of the habitability, survivability, and detectability of life on icy worlds coupled with “Path to Flight” Technology demonstration. Below, we provide a summary of progress (accomplishments) made for each science Investigation.
FY09 was the first year for the Icy Worlds NAI, and as such, it was a year of introductions and beginnings for Education and Public Outreach (E/PO) Program. E/PO staff met with Icy Worlds scientists and with other NAI E/PO leads, to determine how best to design E/PO for this NAI. We established contacts with teachers who are interested in receiving NASA E/PO materials, and began design of astrobiology-related E/PO materials. The highlight of the year was the trip to Barrow, Alaska, where scientists and E/PO leads established contacts at the field research site and met with science teachers and principals at the local schools. Below, we describe our progress in Icy Worlds Education/Public Outreach activities.
Many of our Co-Investigators are actively engaged in planning and development for the prioritized Jupiter Europa Orbiter mission, which will hopefully get a new start from Headquarters in the spring of 2010. PI Kanik is also leading a Discovery study team focusing on a Stardust-style mission to return samples from the plumes of Enceladus.
Investigation 1- Habitability of Icy Worlds Progress
The Habitability Investigation identifies multiple tasks under three categories of objectives pertaining to interiors: Seafloor Processes, Ocean Processes, and Ice Shell Processes. We have made substantial progress in all three areas.
Objective 1: Seafloor Processes
We began testing, through comprehensive laboratory studies, the theory that life emerged ~4 billion years ago within porous and partly permeable submarine, moderate-temperature (≤100°C) alkaline hydrothermal springs. These springs could have occurred on any wet, rocky planet or satellite in our solar system. Using expectations from this theory developed by Co-I Michael Russell, we designed and built a hydrothermal reactor at JPL to simulate hydrothermal vent conditions. We ran the reactor five times and analyzed the solid and fluid products of reactions between carbon dioxide (in oceans) with hydrogen and ammonia (in alkaline hydrothermal solutions). Our preliminary experiments show that HS- and CH4 are produced but not acetate. These results have important implications for life detection on other wet, rocky worlds such Mars and Europa, which require a source of reductants to couple with oxidants produced at their surfaces. This scenario forms an energetic basis for pre-biotic metabolism, as discussed in multiple publications by Dr. Russell and colleagues, and in a review article recently submitted to Nature by Drs. Vance and Hand.
Figure 3.1. Preliminary lab spectra for the BioSpec database. This spectrum is of cultured fungal spores from an Antarctic cryptoendolithic community. Several key spectral features are identified but the spectrum is noisy due to water and interference with the glass slide.
Co-Investigator Steve Vance worked with Lance Christensen of JPL to develop field applications of tunable diode laser absorption spectroscopy for the study of hydrothermal systems. This instrument was used to detect methane production in real time during the last two hydrothermal reactor runs (Figure 1.1). The investigators also successfully identified methyl mercaptan in the laboratory. The system uses the absorption window identified for methane investigations by the Tunable Laser Spectrometer aboard the Mars Science Laboratory, so this new characterization has important implications for imminent life detection measurements at Mars. Drs. Vance and Christensen are now working to identify mercaptan in field samples from The Cedars, a site of active serpentinization in northern California, using their instrument. Their ongoing work will also help to constrain the depth and temperature of serpentinization and test the biogenicity of methane at the Cedars.
Figure 3.2. The snow algae-rich field area in the Sierra Nevada Mountains near Mt. Conness. The red hue of the snow is caused by the pigments of the snow algae C. nivalis.
Figure 3.3. To examine the distribution and ecosystem dynamics of C. nivalis we excavated a trench across one of the snowfields.
Objective 2: Ocean Processes
Co-Investigator Vance developed preliminary salinity inputs to a three-dimensional model for hydrothermal plume dynamics in a europan ocean using FREZCHEM. In order to investigate the effects of salinity and turbulence on material and heat transport processes in icy world oceans,Co-I Goodman and Mr. Lenferink installed a supercomputer system at Wheaton College and implemented a three-dimensional plume dynamics simulation to test theoretical predictions of plume dynamics. First results indicate that mixing of plume and ocean water affects plume temperature, contrary to what prior assumptions. The team will use their models to look next at salinity’s affect on plume dynamics from both a theoretical and computational standpoint.
Figure 3.4. Co-I Dr. Alison Murray uses a sterilized saw to cut out samples from many different depth through the snowpack.
The team embarked on their studies of pre-biotic cell membranes in deep icy world oceans by assembling a fluorescence spectrometer for use with the Simulator for Icy World Interiors in Seattle, and making characterizations of pH-dependent conformation in fatty acid solutions using ANS dye.
Co-Investigators S. Vance, and J. Michael Brown at University of Washington in Seattle, developed a method for encapsulating an aqueous ammonia sample in their optically accessible pressure vessel without concern for contamination by surrounding hydraulic fluid, an important consideration when working with aqueous ammonia and fatty acid solutions. Vance and Brown continued analysis of previously acquired sound velocities in water and magnesium sulfate.
Objective 3: Ice Shell Processes
Co-Investigators Mathieu Choukron and Christophe Sotin, and collaborator Olivier Grasset, examined the creation and stability of clathrate hydrates. Methane clathrates were found to be increasingly unstable with the addition of ammonia to aqueous solutions, to a greater extent than previously expected. Comparison of these new experimental data with thermal models of Titan’s interior suggests a new model for storage and cryovolcanic release of methane on Titan, which is discussed in a forthcoming publication in Icarus. This work is crucial to improving our understanding of exchanges involving volatiles within icy satellites, and the role of clathrates in storing potential light-molecular-weight nutrients and biomarkers.
Figure 3.5. Co-I’s Hand (standing) and Painter (seated with laptop) work together to collect a sequence of spectra across the snowfield surface and down through the trench.
Investigation 2 – Survivability on Icy Worlds Progress
Survivability on icy Worlds investigation examines the survivability of biological compounds under simulated icy world surface conditions, and compares the degradation products to abiotically synthesized compounds resulting from the radiation chemistry on icy worlds.
Co-Investigator Murthy Gudipati and his group focused on studying the photodegradation of PAH molecules in ices using pyrene as the probe. They find that organics are easily ionized and degraded, but unique product formation is not seen in the UV-VIS spectra shown below (see Figures 2.1 and 2.2), indicating that there may be a wide variety of products with no specific absorption features being formed in the ice.
Figure 3.6. Preliminary analysis of our spectra in which we have converted chlorophyll absorption features to cell abundance.
Figure 3.7. The many lakes of the permafrost near Barrow, Alaska. Circles indicate a few of the lakes on which we have begun work.
As a part of Survivability, investigators Paul Johnson, Robert Hodyss and Isik Kanik have begun studying the photo-destruction of organic molecules in icy matrices relevant to solar system bodies. We are measuring the photolytic lifetimes of hydrocarbons in both water and nitrogen matrices. They are also studying the photolytic chemistry of amino acid degradation through matrix isolation experiments. Some of the results are shown in Figures 2.3 and 2.4.
Figure 3.8. Co-I’s John Priscu and Alison Murray working on Ikroavik lake near Barrow, AK.
Figure 3.9. Co-I Adrian Ponce conducts an experiment on wind blown spores along the shore of Ikrovik lake.
Co-I Cooper has been studying the production and sputtering of OH during electron irradiation of ices. One graduate student started working on this project and two undergraduate students are expected to participate soon.
To study the behavior of microbial spores under energetic conditions, Investigators Kevin Hand and Adrian Ponce have installed the monodispersed spores deposition chamber. The irradiation chamber is ready for samples. Dr. Wanwan Yang, a new Caltech postdoctoral fellow, is preparing samples using chamber. We expect samples to be ready for irradiation in 2 months.
Figure 3.10. Airborne spectroscopic data was collected of the Sierra Nevada region as part of our snow algae campaign. The data is from AVIRIS.
Investigation 3 – Detectability on Icy Worlds Progress
Our “Detectability” investigation consists of three high-level Objectives, each of which has several sub-Tasks, many of which are closely linked and interdependent. The Objectives are:
* Objective 1: Detection of Life in the Lab * Objective 2: Detection of Life in the Field * Objective 3: Detection of Life from Orbit
Objective 1: Detection of Life in the Lab
Co-I Hand and Collaborator Carlson have begun work on setting up the BioSpec database, a resource for spectra in the NIR and MIR of microbial isolates. Figure 3.1 shows a preliminary spectrum of cryptoendolithic fungi isolated and cultured by Henry Sun of DRI. We are experimenting with a variety of protocols and procedures so as to yield the best and most reproducible spectra.
Figure 4.1. Deep UV native fluorescence/Raman mapping stage. The scanning area can be as large as 1000cm2 with spatial resolutions from 50 – 3000 um.
Objective 2: Detection of Life in the Field
Two major programs within this objective were initiated during the spring and summer months of 2009. First, our program to spectroscopically map snow algae (primarily C. nivalis)in the Sierra and to understand the underlying biogeochemistry of these ecosystem began with a small field campaign in June. The team, consisting of Co-I’s Kevin Hand (JPL), Tom Painter (Univ. of Utah), and Alison Murray (DRI), along with several students, spent three days mapping, sampling, and excavating snowfields in the Mt. Conness region of the Sierra Nevada (Figure 3.2).
Figure 4.2. Clear ‘sculpter ice’ that has been ultrasonic drilled in several directions to show the
clean holes that can be achieved. These channels will be filled with known concentrations
of specific biochemicals to provide testing of deep UV techniques to characterize the biochemical materials and their spatial distributions as part of the calibration effort to understand the observed UV fluorescence and UV Raman signatures obtained from deep ice cores.
A key question we’re seeking to address is that of why the blooms occur where they do and what controls the onset and distribution of the blooms. By digging a latitudinal trench across an algae-rich snowfield (Figure 3.3) we began work on the depth profile distribution. Co-I Murray collected microbial samples both down and across the transect (Figure 3.4) while Painter and Hand performed both surface and depth-profile spectroscopy of the snowpack and algae (Figure 3.5). The depth-profile spectra will help determine the liquid water distribution with the snow, perhaps a controlling factor over the C. nivalis distribution. We have begun analyzing surface spectra and to date we have a partial map of the algae concentration across the first 2.5 meters of the surface of the transect (Figure 3.6).
Figure 4.3. Deep UV Native fluoresce image of bacterial smears on a background of stainless steel. Each smear is contains approximately 1e6 cells. The image area is 600mm2. The color are representative of cell abundance.
{299}}
Figure 4.5. ESSI/MS5 study of L-arginine (30 ppm in MeOH/H2O) clusters performed using Mini 10.5 MS. Ions at m/z 349 [2Arg+H]+ were isolated from the ion population generated in ESSI from a MeOH/H2O (50% v/v) solution containing 30 ppm L-arginine, 8 ppm caffeine and 60 ppb cocaine. Upon isolation, an ion package (m/z 349) was submitted to the indicated steps of fragmentation and mass selection. Finally, the MS5 product ion mass spectrum was recorded. The symbols indicate the various stages of the MSn experiment.
Our second major initiative is the combined climate-change/spectral biosignatures project to be conducted in the permafrost and lakes of the North Slope Borough region in northern Alaska (Figure 3.7). As part of this work we will be mapping the magnitude and distribution of methane and natural gas release on both local and regional scales across the permafrost and lakes. Our microbiologists and chemist will be exploring the biogenic versus thermogenic nature of this gas release and determine the modern and ancient biogeochemical cycles responsible for the observed signature. A small team including Co-I’s John Priscu (MSU), Alison Murray (DRI), Adrian Ponce (JPL), Rachel Zimmerman-Brachman (JPL), Daniella Scalice (NAI), and Kevin Hand (JPL) began work in some of these lakes around Barrow, AK in mid-August. Rachel and Daniella focused largely on the EPO activities and much detail can be found in the EPO section of this report. Our science team became familiar with operations at the Barrow Arctic Science Consortium and established many important relationships that will help facilitate future work. Samples and data were collected from Ikroavik lake (Figures 3.8 and 3.9) and these will serve as an important baseline for future expeditions that will primarily occur during months when the region is covered in snow and ice.
Figure 1.2.. Seasonally accumulated travertine pools surround an active serpentine spring in the creek running through The Cedars. High concentrations of hydrogen and methane occur in ebullient gases collected from spring sites. A sampling device is shown in the inset. Icy Worlds investigators are developing methods for probing such systems in situ, including rapid detection of life from multiple biomarkers.
Figure 1.5. Results from investigations of Ice Shell Processes indicate an important role for ammonia in determining Titan’s ice shell thickness and the amount of methane within it. a) Generic internal structure model of Titan, not drawn to scale, based on H2O-silicates proportions and thermal evolution (Grasset and Pargamin, Planet. Space Sci., 53, 371-384, 2005; Tobie et al., Icarus, 175, 496-502, 2005). Depths of the interfaces indicated depend on primordial NH3 concentration. b) Pressure-temperature diagram showing the equilibrium thermal profile in a pure H2O warm case (Tobie et al., Nature, 440, 61-64, 2006), the profile across an ice intrusion, and the stability of methane hydrates in the H2O-CH4 system. c) Same as b), for a primordial 5 wt% NH3 concentration. Experimental data are shown for the stability of methane clathrate hydrates obtained here in the H2O-NH3-CH4 with 0%, 5%, and 7.5% NH3.
Objective 3: Detection of Life from Orbit
Though not in the budget or on the schedule for our first year, Co-I’s Hand and Painter and Collaborator Eastwood managed to get time on the Airborne Visible and Infrared Imaging Spectrometer (AVIRIS). Data was collected over the Sierra Nevada mountains in the region where the team had conducted ground based work. The flyover was not at exactly the same as the field campaign, but it was only two weeks later than the fieldwork. The AVIRIS opportunity came at no cost to our NAI project. Figure 3.10 shows the AVIRIS data ‘quick-look’ of the region mapped. We are currently waiting for the calibration files and full data set from the AVIRIS team.
Figure 1.4. Results from a three-dimensional fluid dynamic simulation, illustrated here, test theoretical predictions of hydrothermal plume rise and evolution in Europa’s ocean (Goodman, J. C., Collins, G. C., Marshall, J., and Pierrehumbert, R. T. (2004). Hydrothermal plume dynamics on Europa: Implications for chaos formation. Journal of Geophysical Research-Planets, 109(E3), E03008, doi:10.1029/2003JE002073). These results suggest that mixing plays an important role in plume evolution, contrary to previous assumptions. Ongoing work looks at the effects of salinity on plume dynamics based on theory and simulation.
Investigation 4 – Path to Flight Investigation Progress
The (Field Instrumentation and) Path to Flight investigation’s purpose is to enable in-situ measurements of organics and biological material with field instrumentation that have high potential for future flight instrumentation. The preceding three Investigations provide a variety of measurable goals used to modify or “tune” instrumentation that can be placed in the field. In addition the members of this Investigation provide new measurement capabilities that have been developed with the specific goal of life-detection. The instrument arsenal goes beyond the commercially available instrumentation and brings next generation imaging spectrometers, chromatographic, and sample extraction devices.
The Path to Flight investigation has three (3) primary Objectives, all of which have been progressing well in year 1. Below are the objectives and a list of accomplished tasks.
OBJECTIVE1: Chemistry at Hydrothermal Vents
1. Measurements associated to Laboratory Hydrothermal Vent and other Inv. 1 developments.
OBJECTIVE2: Survivability and Presence in Ice
1. Develop/Re-tune 2D mapping stage for the Deep UV fluorescence/Raman instruments
2. Begin preliminary tests for deployment of deep UV instruments to NICL (National Ice Core Labs).
OBJECTIVE3: Ground Truth and Observability
3. Modification of Instrumentation for deployment in Barrow, Alaska
The following are some additional details associated to the accomplished tasks and preparations for near-term and long-term tasks.
Chemistry at Hydrothermal Vents
Much of the laboratory measurements regarding the custom Hydrothermal Vent reactor are described in Investigation 1. Next steps are include the incorporation of real-time flow instruments that measure the hydrothermal vent fluid in a continuous manner to detect trace amounts of organics that are expected. Some progress has been made along these lines with the application of in situ tunable laser spectrometer to characterize the production of methane. Of particular interest will be the deep UV native fluorescence/Raman instrument with excitation at 248 and 224nm, which has very high sensitivity to organics that contain aromatic rings and reduced carbon species with sensitivity to sulfur and nitrogen bearing organics. In addition a high pressure window is expected to be implemented to the laboratory reactor to accommodate the TUCS and other optical instrument including visible Raman. This will be completed in the Feb – March 2010 timeframe
Survivability and Presence in Ice
Organic and biological detection in ice has traditionally required melting of ice cores resulting in the loss of stratigraphy. Using the deep UV native fluorescence/Raman instruments, referred to as TUCS for Targeted Ultraviolet Chemical Sensors, Co-I’s Arthur Lane and Rohit Bharita with collaborator Claus Mogensen have previously demonstrated the ability to map the distribution of organics and biological material without sample processing; leaving the ice core intact. However, this prototype system used a 2D mapping stage (100 × 20cm) and one of the two motors was faulty and failed in NICL cold room (National Ice Core Labs). The 2D stage has now been refurbished, and reassembled and new custom software by Co-I Rohit Bhartia, collaborator Claus Mogensen, and industry partners Photon Systems, enables autonomous imaging of samples ranging from a couple of millimeters to 2000cm2. In addition new automatic gain adjustment of the TUCS detection system enables 8 orders of magnitude dynamic range from ~10 photons/band to 1e7photons/band, allowing for detection of trace organics (pptrillion) to whole bacterial cells, to communities of bacteria without saturation or loss in signal. Figure 4.1 shows the TUCS on the scanning stage.
Figure 1.1. Results from the laboratory simulation of hydrothermal vents. Five successful reactor runs have been carried out, with which we have shown that (i) HS- can be produced from interaction of hot hydrogen-bearing alkaline solution with metal sulfides, (ii) up to 16 ppmv CH4 is generated from 10 bars of CO2 plus 100 bars H2 (preliminary data) and (iii) no acetate is produced.
In collaboration with Co-I John Priscu, we will be measuring ice cores resident at Montana State, for which some biological analytical assays have been completed. This effort provides a ‘ground-truth’ calibration of UV and UV Raman spectral signatures that can be directly related to specific classes of biological material and biochemical ‘waste’ products that would be formed from insitu biological activity. This effort will follow the initial visit to NICL, and we will bring some sub-sampled pieces of ice core that has shown interesting signatures from the NICL activity to the Priscu facilities at Montana State.
Using 'sculpture’ ice blocks as a surrogate for ice cores, and organically clean ultrasonic drills with melt water aspiration, we have drilled 6 to 8 mm diameter holes 5 to 10 cm deep while maintaining an empty drill hole (Figure 4.2). These serve as test target for the 2D scanner. By inoculating organics (amino acids, fatty acids, proteins) and bacteria within these holes at variable concentrations, we can test sensitivity of the UV fluorescence and UV Raman instruments This data will also serve as the UV signatures library with will support the future Barrow Alaska field work and the scanned ice core studies.
Figure 5.1. Icy Moons trading cards for Education/Public Outreach activities.
Ground Truth and Observability
This area represents the largest collective effort in Investigation 4; however, because of the significant expense involved with field instrumentation development, efforts for leveraged activities are essential. The principal field effort for the Icy Worlds domain is the focus on the Alaska campaigns. Based upon the discussions of the Investigation 3 group in September 2009, the first major campaign is planned to occur over a 7 to 10 day period in late April 2010. Although the scientific theme and principal research areas have not been prioritized for this campaign, it appears that the science team desires to have sample collection capability, in-local-laboratory areas some daily measurement and assessment capability, and several supporting in-situ measurement sets. Based upon their inputs, the following instrumentation is being examined and assessed for their Spring ’10 readiness:
- Subcritical Water Extractor for extracting organic material from various rock/soil samples
- RCAL (Robotic Analytical Chemistry Lab) use especially for detecting iron,
- TUCS (Targeted Ultraviolet Chemical Sensors) and UVBASD: a 1-3m standoff deep UV fluorescence/Raman instrument that was recently developed under Army funding.
- Tunable Laser Diode Spectrometer to examine methane releases from the warming
- Portable Backpack Vis-NIR Reflectance Spectrometer (ASD) to begin to get library
- LUCINA – Mid-IR Microscopic Turbo Fourier Transform Spectrometer for mineral and
There may be a possibility to also utilize a flight-prototype field Raman instrument for small scale characterization of mineral structures on which organic material is detected and to examine wind-blown dust grain that become situated in ice/snow fields and could later become host surfaces and mineral food sources for bacteria.
A second instrument utilization planning meeting should be held in January 2009 to finalize the set of instruments that could be available and the science research tasks that could be reasonably supported with that set.
Progress on Next-Gen instrumentation
Deep UV fluorescence/Raman
Recently, in July 2009, the MMR-SAG presented the strawman payload and specified deep UV native fluorescence/Raman mapping as an instrument for Mars 2018. This instrument provides exquisite detection for organics and provides some degree of chemical specificity with the combination of fluorescence and resonantly enhanced Raman. Co-I Rohit Bhartia has recently demonstrated real-time, label-free, macroscopic and microscopic detection and imaging of single bacterial cells on natural and man-made surfaces using lasers at 224 and 248 nm using ~45nJ at the sample Figure 4.3. The results are being submitted to Science in Nov 2009.
Figure 5.2. Celeste Davis and Rachel LeCover, 2009 summer interns at JPL working on the Icy worlds tasks.
As a part of Investigation 4, we report on the progress of a small light-weight mass spectrometer (MS) capable of chemical analysis of organic material directly from solution or the solid state and with potential value in cryobot missions. The mass spectrometer is handheld and controlled through custom software from a laptop computer. Detection and identification of biosignatures relevant to in situ and remote sensing applications was carried out using the miniature MS equipped with a discontinuous atmospheric pressure interface (DAPI) and a home-built electrosonic spray ionization (ESSI) source. The system was characterized using several target compound classes including biotic and abiotic amino acids, purines, pyrimidines, nucleosides and peptides. Detection limits in the sub-ppm range were achieved with the atmospheric pressure sampling/ionization interface. The results have been submitted to Earth and Planetary Science Letters in October 2009.
Figure 5.4. Life in Extreme Environments Educator Workshop at JPL.
Tandem MS (MS2) was successfully applied to trace detection of target compounds in mixtures. Multiple stage (MSn) analysis, where n=3-5, was employed for molecular structure confirmation and to demonstrate the sensitivity of the instrumentation. The use of this type of mass spectrometer on exploration missions could improve chemical analysis of organic material due to its high sensitivity and specificity, combined with rapid detection and minimal requirements for sample preparation.
Figure 2.1. VUV (10.2 eV) ionization of Pyrene in water ice, while the neutral PAH absorption below 400 nm decreases, ionized PAH absorption increases at around 450 nm. An increase in absorption due to various byproducts can be seen below 300 nm.
we have connections with local schools. He made a presentation to a group of teachers at JPL in January 2009, showing how Alaskan students can use satellite imagery of Barrow to observe changes in climate and other relevant environmental impacts. This will tie in well with the Barrow High School science class on “North Slope Science.” Rachel Zimmerman-Brachman attended a workshop on schoolyard field trips to get ideas for an Icy Worlds NAI schoolyard field trip program.
School Visits/Videoconferencing: Videoconferencing technology was tested between JPL and the Barrow Arctic Science Consortium (BASC) in January 2009, in preparation for future classroom presentations by Icy Worlds scientists. Scientists and students at BASC participated in JPL’s “Life in Extreme Environments” Educator Workshop.
Figure 2.2. Tunable OPO-laser (334, 275, and 235 nm) ionization of Pyrene in water ice. We do not see increase in absorption at any UV-VIS wavelengths. Upon warm-up ionized PAH is partly converted into neutral PAH due to electron-ion recombination.
Website: The Icy Worlds NAI website will be hosted on the NASA Solar System Exploration website beginning in FY10. Currently, astrobiology features on the Solar System Exploration site are posted at http://sse.jpl.nasa.gov/scitech/astrobiology.cfm
Figure 2.3. Photodestruction of glycine in an Ar matrix. The bottom trace shows the infrared spectrum of glycine isolated in Ar. The top trace is the spectrum acquired after 161 minutes of irradiation with a Kr resonance lamp (124nm). Nearly complete destruction of the glycine has occured. The major photolytic mechanism appears to be decarboxylation as evidenced by the CO2 peak at 2342 cm-1 in the upper trace.
Figure 2.4. Photolytic lifetimes of 10% hydrocarbon in N2 ice at 17K, shown as normalized concentration as a function of broadband lamp photolysis time. The half-life of C2H2 was 11.66 minutes, of C2H4 was 16.06 minutes, and of C2H6 was 49.65 minutes. Acetylene is degraded fastest of the three. The flux of our Ar mini-arc broadband lamp was 1 × 1015 photons/cm2s in the 130 to 334 nm range.
Figure 2.5. Microbial survival under simulated Europa radiation conditions. Sown in the top frame are various cycles of the spores. Bottom frame shows the laboratory equipment used for spore culturing, electron irradiation (middle), and analysis processes.
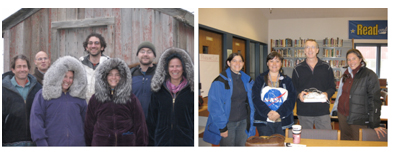
Figure 5.3. Icy Worlds team in Barrow, Alaska; Barrow High School Science Teachers with NAI E/PO Specialists.
Publications
-
Brunetto, R., Pino, T., Dartois, E., Cao, A-T., D’HenDecourt, L., Strazzulla, G., & Bréchignac, P. (2009). Comparison of the Raman spectra of ion irradiated soot and collected extraterrestrial carbon. Icarus, 200(1), 323–337. doi:10.1016/j.icarus.2008.11.004
-
Choukroun, M., Grasset, O., Tobie, G., & Sotin, C. (2010). Stability of methane clathrate hydrates under pressure: Influence on outgassing processes of methane on Titan. Icarus, 205(2), 581–593. doi:10.1016/j.icarus.2009.08.011
-
Ducluzeau, A-L., Van Lis, R., Duval, S., Schoepp-Cothenet, B., Russell, M. J., & Nitschke, W. (2009). Was nitric oxide the first deep electron sink?. Trends in Biochemical Sciences, 34(1), 9–15. doi:10.1016/j.tibs.2008.10.005
-
Hodyss, R., Parkinson, C. D., Johnson, P. V., Stern, J. V., Goguen, J. D., Yung, Y. L., & Kanik, I. (2009). Methanol on Enceladus. Geophysical Research Letters, 36(17), None. doi:10.1029/2009gl039336
-
Martin, W., Baross, J., Kelley, D., & Russell, M. J. (2008). Hydrothermal vents and the origin of life. Nat Rev Micro. doi:10.1038/nrmicro1991
-
Mielke, R. E., Russell, M. J., Wilson, P. R., McGlynn, S. E., Coleman, M., Kidd, R., & Kanik, I. (2010). Design, Fabrication, and Test of a Hydrothermal Reactor for Origin-of-Life Experiments. Astrobiology, 10(8), 799–810. doi:10.1089/ast.2009.0456
-
Nitschke, W., & Russell, M. J. (2009). Hydrothermal Focusing of Chemical and Chemiosmotic Energy, Supported by Delivery of Catalytic Fe, Ni, Mo/W, Co, S and Se, Forced Life to Emerge. Journal of Molecular Evolution, 69(5), 481–496. doi:10.1007/s00239-009-9289-3
-
Sokol, E., Noll, R. J., Cooks, R. G., Beegle, L. W., Kim, H. I., & Kanik, I. (2011). Miniature mass spectrometer equipped with electrospray and desorption electrospray ionization for direct analysis of organics from solids and solutions. International Journal of Mass Spectrometry, 306(2-3), 187–195. doi:10.1016/j.ijms.2010.10.019
-
Strazzulla, G., Garozzo, M., & Gomis, O. (2009). The origin of sulfur-bearing species on the surfaces of icy satellites. Advances in Space Research, 43(9), 1442–1445. doi:10.1016/j.asr.2009.01.007
-
Vance, S., & Michael Brown, J. (2010). Sound velocities and thermodynamic properties of water to 700 MPa and −10 to 100 °C. J. Acoust. Soc. Am., 127(1), 174. doi:10.1121/1.3257223
- Choukroun, M., Grasset, O., Tobie, G. & Sotin, C. (Aug 2009). Stability of methane clathrate hydrates under pressure: Influence on outgassing processes of methane on Titan. Asia-Oceania Geosciences Society 6th Meeting. Singapore.
- Goff-Pochat, Vance, S.,.N. & Collins ., G.C. (2009). Thermal Weathering on Airless Planetary Surfaces. Fall AGU Meeting. San Francisco.
- Goodman, J.C. & Lenferink, E. (2009). Large-eddy Simulations of Europa Hydrothermal Plumes. American Geophysical Union Fall Meeting. San Francisco.
- Gudipati, M. (August 11-15, 2009). Influence of Ice on the Evolution of Solar System Bodies. AOGS International Conference.
- Gudipati, M. (August 11-15, 2009). Radiation Processing of Organics in Solar System Ice Analogs. AOGS International Conference. Singapore.
- Hodyss, R., Johnson, P.V. & Kanik, I. (2010, To be submitted). Photolytic lifetimes of hydrocarbons in water ice matrices. J. Phys Chem.
- Hodyss, R., Johnson, P.V., Boxe, C., Lane, A.L. & Kanik, I. (2009, Submitted.). Fluorescence spectra and quantum yields of aromatic compounds in water ice. Astrobiology.
- Kanik, I., Russell, M.J., Hodyss, R.P. & Johnson, P.V. (14–18 December 2009). Acetate: A better astrobiological indicator of life than methane? AGU Fall Meeting. San Francisco, California, USA.
- Love, G. (June, 2009.). Molecular Fossils and Compound Specific Isotopes. Goldschmidt 2009. Davos, Switzerland.
- Russell, M.J. & Hall, A.J. (2009). A hydrothermal source of energy and materials at the origin of life. In “Chemical Evolution II: From Origins of Life to Modern Society”. American Chemical Society, in press.
- Vance, S., Goff-Pochat, N. & Collins, G.C. (Aug 2009). Thermal Weathering and Erosion on Planetary Surfaces. Asia Oceania Geosciences Symposium. Singapore.
-
PROJECT INVESTIGATORS:
-
PROJECT MEMBERS:
Rohit Bhartia
Co-Investigator
Murthy Gudipati
Co-Investigator
Kevin Hand
Co-Investigator
Arthur Lane
Co-Investigator
Steven Vance
Co-Investigator
-
RELATED OBJECTIVES:
Objective 1.1
Formation and evolution of habitable planets.
Objective 2.1
Mars exploration.
Objective 2.2
Outer Solar System exploration
Objective 3.1
Sources of prebiotic materials and catalysts
Objective 3.2
Origins and evolution of functional biomolecules
Objective 3.3
Origins of energy transduction
Objective 3.4
Origins of cellularity and protobiological systems
Objective 4.1
Earth's early biosphere.
Objective 5.1
Environment-dependent, molecular evolution in microorganisms
Objective 5.3
Biochemical adaptation to extreme environments
Objective 6.1
Effects of environmental changes on microbial ecosystems
Objective 6.2
Adaptation and evolution of life beyond Earth
Objective 7.1
Biosignatures to be sought in Solar System materials
Objective 7.2
Biosignatures to be sought in nearby planetary systems