2013 Annual Science Report
Georgia Institute of Technology
Reporting | SEP 2012 – AUG 2013
Extremophile Ribosomes
Project Summary
Many animals share a common response to environmental stresses. The responses include reorganization of cellular organelles and proteins. Similar stress responses between divergent species suggest that these protective mechanisms may have evolved early and been retained from the earliest eukaryotic ancestors. Many eukaryotic cells have the capacity to sequester proteins and mRNAs into transient stress granules (SGs) that protect most cellular mRNAs. Our observations extend the phylogenetic range of SGs from trypanosomatids, insects, yeast and mammalian cells, where they were first described, to a species of the lophotrochozoan animal phylum Rotifera. We focus on the distribution of three proteins known to be associated with both ribosomes and SG formation: eukaryotic initiation factors eIF3B, eIF4E and T-cell-restricted intracellular antigen 1. We found that these three proteins co-localize to SGs in rotifers in response to temperature stress, osmotic stress and nutrient deprivation as has been described in other eukaryotes. We have also found that the large ribosomal subunit fails to localize to the SGs in rotifers. Furthermore, the SGs in rotifers disperse once the environmental stress is removed as demonstrated in yeast and mammalian cells. These results are consistent with SG formation in trypanosomatids, insects, yeast and mammalian cells, further supporting the presence of this protective mechanism early in the evolution of eukaryotes.
Project Progress
Abstract
Diapausing embryos (resting eggs) from brachionid rotifers are able to withstand desiccation and thermal stress. Resting eggs can remain viable for decades, and develop normally once placed in a permissive environment that allows for hatching, growth and development. The exact mechanisms of resistance are not known, although several molecules have been suggested to confer protection during desiccation and thermal stress. In this study, we have identified by mass spectrometry two thermostable proteins, LEA (late embryogenesis abundant) and VTG (vitellogenin-like), found exclusively in the resting eggs of Brachionus manjavacas. This is the first observation that LEA proteins may play a role in thermostability and the first report of a VTG-like protein in the phylum Rotifera. These proteins exhibited increased expression in rotifer resting eggs when compared to amictic females. Our data suggest the existence of alternate pathways of desiccation and thermal resistance in brachionid rotifers.
Narrative
Encysted embryos of many invertebrates are remarkably resistant to environmental extremes. Desiccation tolerance has been observed in algae, fungi, bacteria and plants, as well as six animal phyla, including nematodes and rotifers (Alpert, 2006). This study focuses on the desiccation tolerance of encysted resting eggs. Resting eggs are able to tolerate desiccation utilizing the processes of diapause or anhydrobiosis (Clegg, 2001; Keilin, 1959; Ricci, 2001). Diapause is a state of low metabolic activity, typically associated with both arrested development and environmental extremes (reviewed in Schroder, 2005). Diapause is developmentally programmed in plants, rotifers, daphnids, copepods, and Artemia (Clark et al., 2012). In contrast, anhydrobiosis is a period in which metabolism is suppressed due to environmental extremes that are unfavorable for both growth and reproduction. Anhydrobiosis can occur during any life stage while diapause typically occurs during embryonic development (Caceres, 1997; Clark et al., 2012; Keilin, 1959).
The ability of anhydrobiotic organisms to survive in a desiccated state is associated with an increase in the viscosity of the cytoplasm (Buitink and Leprince, 2004). The increase in viscosity of the cytoplasm is caused by an increase in the amounts of sucrose and oligosaccharides within the cytoplasm. The increased sugar content causes the cytoplasmic fluid to become more glass-like. The glass-like fluid forms in large part due to the concentration of sucrose and oligosaccharide within the cytoplasm (reviewed in Berjak, 2006). These sugars appear to be tightly associated with specific heat soluble proteins (Walters et al., 1997).
The encysted embryos of Artemia franciscana (brine shrimp) have been found in an astounding range of extreme environments (Warner et al., 2004), and have a documented tolerance of hypersaline waters, high doses of ultraviolet radiation, anoxia, and temperature extremes (Alpert, 2006). Large amounts of two proteins, p26 and artemin, are produced in A. franciscana encysted embryos in response to environmental stresses. p26 is a heat shock protein and a proposed molecular chaperone. Artemin is a thermally stable RNA binding protein, and has also been implicated as a possible molecular chaperone (Sharon et al., 2009; Warner et al., 2004). Current research suggests that molecular chaperones prevent protein aggregation in encysted embryos during stressful environmental conditions (Sharon et al., 2009). Much research has been devoted to explaining the cellular and molecular basis for environmental stress tolerance in A. franciscana encysted embryos, but little research has explored the molecular mechanisms by which encysted monogonont rotifer embryos are able to withstand similar environmental stressors.
Monogonont rotifers are aquatic micro-invertebrates that have a cyclical parthenogenetic life cycle (Wallace et al., 2006). Sexual reproduction produces diapausing embryos: resting eggs that are resistant to multiple environmental stressors. The resting eggs of Brachionus species are able to withstand extreme temperatures, desiccation, and ionizing radiation (Gladyshev and Meselson, 2008; Hagiwara et al., 1995; Kotani et al., 2001; Schroder, 2005). After air drying, resting eggs typically contain 70% water, but remain viable after freeze-drying to only 7% water (Balompapueng et al., 1997; Hagiwara, 1996). Resting eggs in the sediments of lakes, ponds and coastal lagoons are able to remain viable for decades and resume normal growth and development once the environment becomes suitable for growth (Alpert, 2005; Denekamp et al., 2011; Garcia-Roger et al., 2006; Gilbert and Schroder, 2004; Hagiwara et al., 2005; Kotani et al., 2001; Minkoff et al., 1983). The exact mechanisms by which the rotifer resting eggs are able to survive extreme environments remain unknown.
Up-regulation of a number of late embryogenesis abundant (LEA) genes has been documented in both plants and animals during desiccation (Warner et al., 2004). LEA proteins have been implicated in various functions including molecular shields, chaperones, membrane protectants, ion sinks, hydration buffers, and antioxidants (Battaglia et al., 2008; Shih et al., 2008; Tunnacliffe and Wise, 2007). LEA proteins enable plants, certain prokaryotes, and a small number of animal species, to survive both desiccation and temperature extremes (Goyal et al., 2005a; Hand et al., 2007; Hand et al., 2011; Koltai et al., 2004; Sharon et al., 2009; Wolkers et al., 2001). LEA proteins have been proposed to protect cellular and molecular structures from damage induced by both low temperatures and desiccation in plants (Tunnacliffe and Wise, 2007; Wise, 2003; Wolkers et al., 2001). While the initial characterization of LEA proteins was completed in plants, more recent studies have also found them in nematodes, prokaryotes and brine shrimp (Battista et al., 2001; Berjak, 2006; Dure and Galau, 1981; Koltai et al., 2004; Sharon et al., 2009; Wise, 2003; Wolkers et al., 2001).
Other molecules, including the disaccharides trehalose and sucrose, have also been identified as contributors to the ability of plants and animals to survive desiccation (Crowe et al., 1998; Hoekstra et al., 2001).
Recent studies show an apparent lack of non-reducing disaccharides in some desiccation resistant organisms; specifically, two desiccation tolerant bdelloid rotifers, Philodina roseola and Adineta vaga, are tolerant to desiccation but lack trehalose synthase genes (Atapour and Moharramipour, 2009; Hengherr et al., 2008; Lapinski and Tunnacliffe, 2003). These data supports the theory that multiple complementary pathways exist for the adaptation of organisms to harsh environments; however, not much is known about what these pathways might be. Understanding adaptive mechanisms, which enable organisms to survive extreme environments, could provide insight into the mechanisms by which life may have survived on the early earth and may survive extraterrestrially.
In this study we begin to investigate the mechanisms by which Brachionus spp. are able to tolerate extreme environmental challenges. Molecular mechanisms protecting rotifers from environmental stresses most likely exist because the resting eggs of brachionid rotifers are able to withstand the environmental extremes of desiccation and high temperatures. We have identified two proteins that may contribute to their resistance, including one similar to a LEA protein already described in rotifers and a second similar to the vitellogenin protein in chicken egg yolk (Denekamp et al., 2009; Hartling et al., 1997; Piulachs et al., 2003). Substantial amounts of both proteins are present in resting eggs, but are absent in adult rotifer females.
Resting eggs of brachionid rotifers are able to survive desiccation without difficulty. Within 15-30 hours following rehydration, the rotifers transition from a desiccated state in which no protein synthesis occurs to one of full metabolic activity. More than 20 percent of the B. calyciflorus and B. manjavacas resting eggs hatched after 19 hours of hydration (Figure 1). In contrast, B. rotundiformis resting eggs hatched after 30 hours following hydration. Desiccated adults of the bdelloid rotifers A. vaga and P. rapida displayed movement in as little as 15 minutes of rehydration (data not shown).
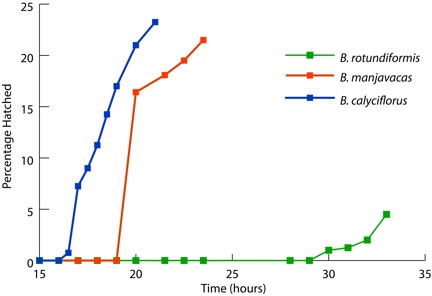
The heat tolerance of active adults, desiccated resting eggs (brachionids), or desiccated adults (bdelloids) was evaluated. Adults of B. calyciflorus, A. vaga and P. rapida were unable to survive temperatures greater than 40°C for one hour (Figure 2). In contrast, desiccated B. calyciflorus resting eggs, and A. vaga and P. rapida desiccated adults, were able to survive exposure to temperatures exceeding 90°C for one hour.

B. manjavacas thermostable proteins were isolated by heating cell lysate from active adults or resting eggs at 70°C for seven minutes. Proteins resistant to denaturation at this temperature failed to aggregate and remained in the soluble fraction after centrifugation. SDS PAGE followed by Coomassie staining visualized these proteins (Figure 3).

Heating adult female lysate yielded no major heat-stable proteins (Figure 3, Lane 2). In contrast, heating lysate from desiccated resting eggs yielded two major heat-stable proteins with masses of 35 kD and 27 kD (Figure 3, Lane 4). These proteins were subjected to tryptic digest and mass spectrometry. Analysis of the 35 kD protein resulted in twenty-two total spectra, 11 unique spectra and 11 unique peptides (Figure 4). From the 35 kD protein, 11 unique peptides had 100% identity with vitellogenin, a yolk protein in chickens. However, these peptides cover only about 9 % of the full vitellogenin sequence, so more data are required for definitive protein identification.

Analysis of the 27 kD protein resulted in 2 total and unique spectra, six peptide sequences, and two unique peptides (Figure 5). The six peptides had 100% identity with LEA-1B from the bdelloid rotifer Adineta vaga (Gladyshev and Arkhipova, 2010).

Western blotting confirmed the expression of LEA and VTG-like proteins in adult females and resting eggs of B. manjavacas. The VTG-like protein was expressed in both resting eggs and adult females. However, its expression was higher in resting eggs (Figure 6a). A LEA-like protein was expressed in resting eggs, however, it was not expressed in adult females (Figure 6b). To our knowledge, this is the first description of a VTG-like protein in the phylum Rotifera and also the first characterization of a LEA-like protein as thermostable in a brachionid species.

VTG-like and LEA-like gene isolation
Our initial strategy involved designing degenerate primers based upon the peptide sequences determined through mass spectrometry. The degenerate primers contained all codon possibilities for the peptide sequences. We first attempted to amplify the gene using genomic DNA as template. This strategy proved to be unsuccessful, most likely due to the existence of introns within the genomic DNA. Next, we used mRNA from desiccated resting eggs as the template in a reverse transcription-PCR reaction (RT-PCR). To eliminate complications from introns, cDNA from desiccated resting eggs were also used as template to amplify the VTG-like and LEA-like thermostable protein genes; however, this strategy was also unsuccessful. To date we have attempted the gene amplification reactions with freshly produced, non-desiccated resting eggs, resting egg bearing females, mictic females and amictic females. All attempts were unsuccessful.
Brachionid rotifers are adapted to environments where their growth is episodically restricted by harsh environments (Denekamp et al., 2009). As a result, the production of resting eggs ensures rotifer survival through periods unfavorable for population growth. Encysted rotifer embryos are able to survive a number of environmental extremes including temperature, salinity, anoxia, UV, and desiccation and remain viable for decades (Hagiwara et al., 1995; Kotani et al., 2001; Schroder, 2005). Several adaptations have been suggested to explain the ability of organisms to survive environmental stresses, particularly trehalose, sucrose and class 3 LEA proteins (Boschetti et al., 2011; Crowe et al., 1998; Hand et al., 2011; Hoekstra et al., 2001).
In this study, we demonstrated that metabolic activity in desiccated resting eggs of the Brachionus spp. resumes within hours after hydration. Nearly 25% of dormant resting eggs from both B. manjavacas and B. calyciflorus were able to hatch within 16 hours of hydration. This work also demonstrated that desiccated diapaused resting eggs and anhydrobiotic adult rotifers remain viable after being subjected to extreme heat, whereas active adult rotifers do not.
Our work identified VTG-like and LEA-like proteins in B. manjavacas that may play essential roles during both desiccation and thermal stress. These two thermostable proteins were identified in resting eggs, and may contribute to heat resistance. Our finding of increased expression of the LEA-like protein in desiccated Brachionus resting eggs is supported by previous studies with other animal models. LEA proteins have also been isolated in chironomids, bdelloid rotifers, nematodes and tardigrades. These are anhydrobiotic organisms, which display an upregulation of LEA proteins during the period of desiccation (Kikawada et al., 2006; Pouchkina-Stantcheva et al., 2007; Reardon et al., 2010; Sharon et al., 2009). Previous studies have also identified high expression of several groups of LEA genes in resting eggs and females carrying resting eggs in Brachionus (Denekamp et al., 2011; Denekamp et al., 2010; Denekamp et al., 2009). However, this study has identified a type of LEA protein previously undescribed from brachionid rotifers. The LEA-like peptides isolated in this study have 95% identity with both the LEA-1A and LEA-1B proteins identified in the bdelloid rotifer, Adineta ricciae. Denekamp et al. have previously identified two group 3 LEA proteins in Brachionus plicatilis and have implicated one of the LEA proteins as being heat stable (Denekamp et al., 2010; Denekamp et al., 2009). The LEA-like peptides isolated in this study have no similarity with the group 3 LEA proteins identified in Brachionus plicatilis by Denekamp and colleagues (Denekamp et al., 2010; Denekamp et al., 2009).
Vitellogenin is the major yolk protein found in fish, amphibians, reptiles, birds and many invertebrates (Piulachs et al., 2003). We report here the first isolation of a vitellogenin-like protein in the phylum Rotifera. While the highest match attained for the vitellogenin-like protein is against the vitellogenin from Gallus gallus (chicken), there were also other matches against vitellogenins found in Meleagris gallopavo (wild turkey), Coturnix japonica (Japanese quail), Taeniopygia guttata (zebra finch), Anolis carolinensis (lizard) and Larus argentatus (Herring gull). There were no matches to vitellogenin proteins found in any more closely related species.
Previous work with vitellogenin in flounders supports our observation that vitellogenin may contribute to the heat resistance of embryos (Hartling et al., 1997). Strong expression in resting eggs with little to no expression of both proteins in females suggests that expression of these genes is induced during the development of the resting eggs.
Despite multiple attempts, we were unable to isolate the VTG-like and LEA-like gene sequences from B. manjavacas. Our results suggest that the mRNA message is present only in specific developmental stages. The proteins are likely to be more stable and able to persist in desiccated resting eggs. In contrast, the mRNA message is probably no longer present or present in very small amounts in desiccated resting eggs. In contrast, we were able to amplify the group three LEA genes using the primers described in Denekamp et al. (Denekamp et al., 2010).
Denekamp et al. generated expressed sequence tags (ESTs) in an attempt to characterize the genes expressed during formation of resting eggs, dormant resting eggs, and hatching resting eggs (Denekamp et al., 2009). The study produced an assembly of 18, 000 putative transcripts. Three transcripts matching group 3 LEA proteins were present in the ESTs; however, none were similar to the LEA-like protein isolated through our analysis of thermostable proteins. No VTG-like transcript was identified in the EST analysis (Denekamp et al., 2009). In fact, the authors proposed that another protein, lipoprotein lipase, functions as the yolk protein in resting eggs, not a VTG-like protein. The data from the Denekamp study is consistent with our hypothesis that both the VTG-like and LEA-like genes are expressed only at discrete developmental stages. The greatest obstacle to successful isolation of these genes appears to be the inability to isolate enough mRNA from specific developmental stages.
Future studies need to verify the function of the LEA-like and VTG-like proteins, possibly including activity as molecular chaperones. Goyal et al. have proposed that LEA proteins are able to prevent aggregation of cellular proteins by preventing interactions between partially unfolded proteins (Goyal et al., 2005a; Goyal et al., 2005b). Hand and colleagues have suggested that LEA proteins most likely prevent interactions by serving as molecular chaperones (Hand et al., 2011).
In summary, we isolated both VTG-like and LEA-like thermostable proteins from the rotifer B. manjavacas that are present primarily in resting eggs. Our data suggests that these proteins may be important to the survival of encysted rotifer embryos during environmental stress like desiccation and high temperature. To our knowledge, this is the first description of a VTG-like protein in the phylum Rotifera and also the first characterization of a LEA-like protein as thermostable in a brachionid species.
References
Alpert, P., 2005. The limits and frontiers of desiccation-tolerant life. Integrative and comparative biology 45, 685-695.
Alpert, P., 2006. Constraints of tolerance: why are desiccation-tolerant organisms so small or rare? J Exp Biol 209, 1575-1584.
Altschul, S.F., Gish, W., Myers, E.W., Lipman, D.J., 1990. Basic local alignment search tool. J Mol Biol 215, 403-410.
Atapour, M., Moharramipour, S., 2009. Changes of cold hardiness, supercooling capacity, and major cryoprotectants in overwintering larvae of Chilo suppressalis (Lepidoptera: Pyralidae). Environmental entomology 38, 260-265.
Balompapueng, M.D., Hagiwara, A., Nozaki, Y., Hirayama, K., 1997. Preservation of resting eggs of the euryhaline rotifer Brachionus plicatilis O. F. Müller by canning. Hydrobiologia 358, 163-166.
Battaglia, M., Olvera-Carrillo, Y., Garciarrubio, A., Campos, F., Covarrubias, A.A., 2008. The enigmatic LEA proteins and other hydrophilins. Plant Physiol 148, 6-24.
Battista, J.R., Park, M.-J., McLemore, A.E., 2001. Inactivation of two homologues of proteins presumed to be involved in desiccation tolerance of plants sensitizes Deinococcus radiodurans R1 to desiccation. Cryobiology 43, 133 – 139.
Berjak, P., 2006. Unifying perspectives of some mechanisms basic to desiccation tolerance across life forms. Seed Sci Res 16, 1-15.
Boschetti, C., Pouchkina-Stantcheva, N., Hoffmann, P., Tunnacliffe, A., 2011. Foreign genes and novel hydrophilic protein genes participate in the desiccation response of the bdelloid rotifer Adineta ricciae. J Exp Biol 214, 59-68.
Buitink, J., Leprince, O., 2004. Glass formation in plant anhydrobiotes: survival in the dry state. Cryobiology 48, 215-228.
Caceres, C.E., 1997. Temporal variation, dormancy, and coexistence: a field test of the storage effect. Proceedings of the National Academy of Sciences of the United States of America 94, 9171-9175.
Clark, M.S., Denekamp, N.Y., Thorne, M.A., Reinhardt, R., Drungowski, M., Albrecht, M.W., Klages, S., Beck, A., Kube, M., Lubzens, E., 2012. Long-Term Survival of Hydrated Resting Eggs from Brachionus plicatilis. PLoS One 7, e29365.
Clegg, J.S., 2001. Cryptobiosis—a peculiar state of biological organization. Comparative biochemistry and physiology. Part B, Biochemistry & molecular biology 128, 613-624.
Crowe, J.H., Carpenter, J.F., Crowe, L.M., 1998. The role of vitrification in anhydrobiosis. Annu Rev Physiol 60, 73-103.
Denekamp, N., Reinhardt, R., Albrecht, M., Drungowski, M., Kube, M., Lubzens, E., 2011. The expression pattern of dormancy-associated genes in multiple life-history stages in the rotifer Brachionus plicatilis. Hydrobiologia 662, 51-63.
Denekamp, N.Y., Reinhardt, R., Kube, M., Lubzens, E., 2010. Late embryogenesis abundant (LEA) proteins in nondesiccated, encysted, and diapausing embryos of rotifers. Biol Reprod 82, 714-724.
Denekamp, N.Y., Thorne, M.A., Clark, M.S., Kube, M., Reinhardt, R., Lubzens, E., 2009. Discovering genes associated with dormancy in the monogonont rotifer Brachionus plicatilis. BMC Genomics 10, 108.
Dure, L., Galau, G.A., 1981. Developmental Biochemistry of Cottonseed Embryogenesis and Germination : XIII. Regulation of Biosynthesis of principal storage proteins. Plant Physiol 68, 187-194.
Garcia-Roger, E., Carmona, M., Serra, M., 2006. Patterns in rotifer diapausing egg banks: Density and viability. Journal of Experimental Marine Biology and Ecology 336, 198 -210.
Gilbert, J.J., Schroder, T., 2004. Rotifers from diapausing, fertilized eggs: unique features and emergence. Limnology and Oceanography 49, 1341-1354.
Gladyshev, E., Meselson, M., 2008. Extreme resistance of bdelloid rotifers to ionizing radiation. Proceedings of the National Academy of Sciences of the United States of America 105, 5139-5144.
Gladyshev, E.A., Arkhipova, I.R., 2010. Genome Structure of Bdelloid Rotifers: Shaped by Asexuality or Dessication? Journal of Heredity 101, S85-S93.
Goyal, K., Pinelli, C., Maslen, S.L., Rastogi, R.K., Stephens, E., Tunnacliffe, A., 2005a. Dehydration-regulated processing of late embryogenesis abundant protein in a desiccation-tolerant nematode. FEBS Lett 579, 4093-4098.
Goyal, K., Walton, L.J., Tunnacliffe, A., 2005b. LEA proteins prevent protein aggregation due to water stress. Biochem J 388, 151-157.
Guillard, R.R., Ryther, J.H., 1962. Studies of marine planktonic diatoms. I. Cyclotella nana Hustedt, and Detonula confervacea (cleve) Gran. Can J Microbiol 8, 229-239.
Hagiwara, A., 1996. Use of resting eggs for mass preservation of marine rotifers. Technical Reports of Japanese Sea Ranching Programs 24, 109 – 120.
Hagiwara, A., Hoshi, N., Kawahara, F., Tominaga, K., Hirayama, K., 1995. Resting eggs of the marine rotifer Brachionus plicatilis Muller: development, and efect of irradiation on hatching Hydrobiologia 313/314, 223-229.
Hagiwara, A., Kadota, Y., Hino, A., 2005. Maternal effect by stem females in Brachionus plicatilis: effect of starvation on mixis induction in offspring. Hydrobiologia 546, 275-279.
Hand, S.C., Jones, D., Menze, M.A., Witt, T.L., 2007. Life without water: expression of plant LEA genes by an anhydrobiotic arthropod. Journal of experimental zoology. Part A, Ecological genetics and physiology 307, 62-66.
Hand, S.C., Menze, M.A., Toner, M., Boswell, L., Moore, D., 2011. LEA proteins during water stress: not just for plants anymore. Annu Rev Physiol 73, 115-134.
Hartling, R.C., Pereira, J.J., Kunkel, J.G., 1997. Characterization of a heat-stable fraction of lipovitellin and development of an immunoassay for vitellogenin and yolk protein in winter flounder (Pleuronectes americanus). J Exp Zool 278, 156-166.
Hengherr, S., Heyer, A.G., Kohler, H.R., Schill, R.O., 2008. Trehalose and anhydrobiosis in tardigrades—evidence for divergence in responses to dehydration. The FEBS journal 275, 281-288.
Higgins, D.G., BLeasby, A.J., Fuchs, R., 1992. Clustal V: improved software for multile sequence alignment. Computer Applications in the Biosciences (CABIOS) 8, 189 – 191.
Hoekstra, F.A., Golovina, E.A., Buitink, J., 2001. Mechanisms of plant desiccation tolerance. Trends Plant Sci 6, 431-438.
Juris, S.J., Shah, K., Shokat, K., Dixon, J.E., Vacratsis, P.O., 2006. Identification of otubain 1 as a novel substrate for the Yersinia protein kinnase using chemical genetics and mass spectrometry. FEBS Lett 580, 179-183.
Keilin, D., 1959. The problem of anabiosis or latent life: history and current concept. Proc R Soc Lond B Biol Sci 150, 149-191.
Kikawada, T., Nakahara, Y., Kanamori, Y., Iwata, K., Watanabe, M., McGee, B., Tunnacliffe, A., Okuda, T., 2006. Dehydration-induced expression of LEA proteins in an anhydrobiotic chironomid. Biochemical and biophysical research communications 348, 56-61.
Koltai, H., Gal, T.Z., Glazer, I., 2004. An LEA group 3 family member is involved in survival of C-elegans during exposure to stress. FEBS Lett 577, 21-26.
Kotani, T., Ozaki, M., Matsuoka, K., Snell, T.W., Hagiwara, A., 2001. Reproductive isolation among geographically and temporally isolated marine Brachionus strains. Hydrobiologia 446, 283-290.
Laemmli, U.K., 1970. Cleavage of structural proteins during the assembly of the head of bacteriophage T4. Nature 227, 680-685.
Lapinski, J., Tunnacliffe, A., 2003. Anhydrobiosis without trehalose in bdelloid rotifers. FEBS Lett 553, 387-390.
Minkoff, G., Lubzens, E., Kahan, D., 1983. Environmental factors affecting hatching of rotifer (Brachionus plicatilis) resting eggs. Hydrobiologia 104, 61-69.
Nichols, Stein, 1973. Growth media—freshwater, in: J.R. Stein (Ed.), Handbook of Phycological Methods. Cambridge University Press, Cambridge, 7-24.
Piulachs, M.D., Guidugli, K.R., Barchuk, A.R., Cruz, J., Simoes, Z.L., Belles, X., 2003. The vitellogenin of the honey bee, Apis mellifera: structural analysis of the cDNA and expression studies. Insect biochemistry and molecular biology 33, 459-465.
Pouchkina-Stantcheva, N.N., McGee, B.M., Boschetti, C., Tolleter, D., Chakrabortee, S., Popova, A.V., Meersman, F., Macherel, D., Hincha, D.K., Tunnacliffe, A., 2007. Functional divergence of former alleles in an ancient asexual invertebrate. Science 318, 268-271.
Preston, B., Snell, T., Dingman, B., 2000. Use of the freshwater rotifer Brachionus calyciflorus in a screening assay for potential endocrine disruptors. Environmental Toxicology and Chemistry 19, 2923 – 2928.
Reardon, W., Chakrabortee, S., Pereira, T.C., Tyson, T., Banton, M.C., Dolan, K.M., Culleton, B.A., Wise, M.J., Burnell, A.M., Tunnacliffe, A., 2010. Expression profiling and cross-species RNA interference (RNAi) of desiccation-induced transcripts in the anhydrobiotic nematode Aphelenchus avenae. BMC molecular biology 11, 6.
Ricci, C., 2001. Dormancy patterns in rotifers. Hydrobiologia 446/447, 1-11.
Schroder, T., 2005. Diapause in monogonont rotifers. Hydrobiologia 546, 291 – 306.
Sharon, M.A., Kozarova, A., Clegg, J.S., Vacratsis, P.O., Warner, A.H., 2009. Characterization of a group 1 late embryogenesis abundant protein in encysted embryos of the brine shrimp Artemia franciscana. Biochem Cell Biol 87, 415-430.
Shih, M.-d., Hoekstra, F.A., Hsing, Y.-I.C., 2008. Chapter 4 Late Embryogenesis Abundant Proteins, in: K. Jean-Claude, D. Michel (Eds.), Advances in Botanical Research. Academic Press, 211-255.
Stout, E.P., La Clair, J.J., Snell, T.W., Shearer, T.L., Kubanek, J., 2010. Conservation of progesterone hormone function in invertebrate reproduction. Proceedings of the National Academy of Sciences of the United States of America 107, 11859-11864.
Thompson, J.D., Higgins, D.G., Gibson, T.J., 1994. Clustal W: improving sensiticity of progressive multiple sequence alignment through sequence weighting, position-specific gap penalties and weight matrix choice. Nucleic Acids Research 22, 4673 – 4680.
Tunnacliffe, A., Wise, M.J., 2007. The continuing conundrum of the LEA proteins. Die Naturwissenschaften 94, 791-812.
Wallace, R.L., Snell, T.W., Ricci, C., Nogrady, T., 2006. Part 1: Biology, Ecology and Systematics. Guides to the Identification of Microinvertebrates of the Continental Waters of the World. Backhuys Publishers, Ghent, The Netherlands.
Walters, C., Ried, J.L., Walker-Simmons, M.K., 1997. Heat-soluble proteins extracted from wheat embryos have tightly bound sugarsand unusual hydration properties. Seed Sci Res 7, 125 – 134.
Warner, A.H., Brunet, R.T., MacRae, T.H., Clegg, J.S., 2004. Artemin is an RNA-binding protein with high thermal stability and potential RNA chaperone activity. Arch Biochem Biophys 424, 189-200.
Wise, M.J., 2003. LEAping to conclusions: A computational reanalysis of late embryogenesis abundant proteins and their possible roles. Bmc Bioinformatics 4.
Wolkers, W.F., McCready, S., Brandt, W.F., Lindsey, G.G., Hoekstra, F.A., 2001. Isolation and characterization of a D-7 LEA protein from pollen that stabilizes glasses in vitro. Biochimica et biophysica acta 1544, 196-206.
Publications
-
Jones, B. L., Schneider, D. M., & Snell, T. W. (2012). Thermostable proteins in the diapausing eggs of Brachionus manjavacas (Rotifera). Comparative Biochemistry and Physiology Part A: Molecular & Integrative Physiology, 162(3), 193–199. doi:10.1016/j.cbpa.2012.02.020
-
Jones, B. L., VanLoozen, J., Kim, M. H., Miles, S. J., Dunham, C. M., Williams, L. D., & Snell, T. W. (2013). Stress granules form in Brachionus manjavacas (Rotifera) in response to a variety of stressors. Comparative Biochemistry and Physiology Part A: Molecular & Integrative Physiology, 166(2), 375–384. doi:10.1016/j.cbpa.2013.07.009
-
PROJECT INVESTIGATORS:
-
PROJECT MEMBERS:
Brande Jones
Co-Investigator
Shreyas Athavale
Collaborator
Loren Williams
Collaborator
-
RELATED OBJECTIVES:
Objective 3.2
Origins and evolution of functional biomolecules
Objective 4.2
Production of complex life.
Objective 5.3
Biochemical adaptation to extreme environments