2012 Annual Science Report
University of Wisconsin
Reporting | SEP 2011 – AUG 2012
Project 1B: Extracellular Polymeric Substances (EPS) and Bacterial Toxicity of Oxides
Project Summary
Our interdisciplinary project examined the hypotheses that (1) bacterial cell membranes are ruptured in contact with specific mineral surfaces, (2) biofilm-forming extra-cellular polymeric substances (EPS) may have evolved to shield against membrane rupture (cell lysis), (3) differences in cell-wall structure of Gram-negative and Gram-positive bacteria may influence the susceptibility of cells to toxic minerals and (4) mineral toxicity depends on its surface chemistry and nanoparticle size.
We have confirmed that the viability of wild-type bacterial cells which make EPS and biofilm is higher than that of mutant-type bacteria when exposed to oxide minerals. The effect is seen for both Gram negative and Gram positive bacteria, where the latter are less susceptible to the toxicity of minerals (Xu et al., 2012, Astrobiology; Zhu et al., in prep.). Thus, the thicker peptidoglycan outermost layer of the Gram positive cell surface provides an additional layer of protection compared to the less rigid, phospholipid outer membrane of the Gram negative bacteria (Zhu et al., in prep.). Most interestingly, EPS production could be induced by exposure to toxic minerals. The toxicity of the minerals depends on their surface chemistry (surface charge, ability to generate photocatalytic reactive oxygen species (ROS)) and size (Xu et al., in review).
By understanding the mechanisms for membranolysis, especially under the extreme conditions of high radiation and heavy impacts during early planetary history, the project addresses the NASA Astrobiology Institute’s (NAI) Roadmap goals of understanding the origins of cellularity, the evolution of mechanisms for survival at environmental limits, and preservation of biosignatures, and NASA’s Strategic Goal of advancing scientific knowledge of the origin and evolution of the Earth’s biosphere and the potential for life elsewhere.
Project Progress
We used whole bacterial cells to address the question of whether EPS provides armor against cell lysis. A Gram negative wild-type bacterium, Pseudomonas aeruginosa PAO1, which is a robust EPS producer and its knock out mutant, Δ-psl , with defective ability to form EPS and biofilm were used to conduct cell viability studies in suspensions of oxide particles with cells (Figure 1). Wild type cell viability was greater than that of the mutant type. Furthermore, viability was oxide-dependent, increasing as γ-Al2O3 > amorphous SiO2 (Figure 2). Thus, our results confirm our hypothesis that EPS acts as a shield against the toxicity of certain minerals.
We also examined the cell + oxide particle suspensions using High Resolution Transmission Electron Microscopy (HRTEM) to visualize the location of the particles with respect to the cell surface (Figure 3). The amorphous silica particles were located in the extra-cellular region of both wild type cells that possess EPS as well as washed mutant type cells that lack EPS, suggesting that the similar negative charge on both SiO2 particles and the cell surfaces resulted in electrostatic repulsion and prevented cell-particle contact. In contrast, γ -Al2O3 particles of size < 80 nm were seen inside the washed mutant type cells, but were seen only in the extracellular space for the wild type cells. These results indicated that the positively-charged γ-Al2O3 particles were electrostatically attracted to the oppositely-charged cell surface. The particles could not penetrate in the case of the wild type due to the presence of the EPS, whereas the smaller particles < 80 nm could enter the mutant type cells that lack EPS.
The toxicity of anatse even without entering the cell was explained by the presence of photocatalytically induced reactive oxygen species (ROS) at the titania surface. ROS can oxidize organic molecules and are known to be membranolytic to cells. The reactivity of these species can be quenched, however, by specific enzymes which scavenge these free radicals. Thus, the relative viability of cells in the presence of bovine liver catalase-treated anatase was much higher than in the case of anatase alone (Figure 4).
Furthermore, we showed that EPS does not simply act as a passive barrier to nanoparticle toxicity. The surface charge of alumina particles was reversed from positive to negative by the adsorption of EPS generated by the wild-type cells, such that repulsion with the negatively-charged surfaces reduced the toxicity of alumina compared to that of mutant type cells which could not generate EPS. Most significantly, the presence of the toxic nanoparticles could induce the formation of additional EPS by the wild type cells compared to the particle-free blanks (Figure 5).
We also determined if the difference in cell wall structure of Gram negative versus Gram positive bacteria could influence the effects of EPS and nanoparticle toxicity. We used biofilm-producing wild-type, NCIB3610, and biofilm-defective mutant, yhxBΔ. We confirmed that the presence of EPS does protect Gram-positive bacteria from toxic oxides. Oxide toxicity was also specific to the mineral in the case of the Gram positive bacteria (Figure 6). Thus, our hypothesis that EPS may have evolved early in the evolution of life seems to hold across the two bacterial types. In addition, the Gram positive bacteria are slightly less susceptible to nanoparticle toxicity compared to the Gram negative bacteria. We interpret this result to indicate that the thick outer layer of peptidoglycan compared to the thin peptidoglycan layer of Gram negative bacteria provides additional protection against the toxicity of oxides (Figure 6).
Our results support our hypotheses that EPS acts an armor against mineral toxicity, cell wall structure differences also provide additional armor and that not all minerals are toxic or benign, but that toxicity depends on mineral properties such as surface charge, presence of surface free radicals and particle size.
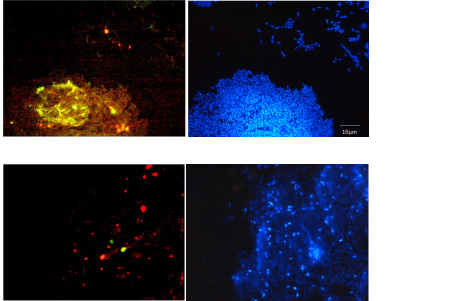
Fluorescent lectin stains (green and red) confirming presence of EPS and biofilm and DNA stain (blue) to localize bacterial cells with respect to the biofilm. Note presence of EPS and biofilm for the Gram negative wild type P. aeruginosa PAO1 bacteria (upper panel) and limited EPS and biofilm for the knock-out mutant type, del-psl (lower panel).
Viability of Gram negative wild type and mutant type cells in the presence of various oxides relative to cell viability corresponding oxide-free controls. Note mutant type is more susceptible than wild type confirming the protective role of EPS against mineral toxicity. Also, not all minerals are equally toxic.
High Resolution Transmission Electron Microscope images indicating the localization of oxide particles with respect to bacterial cell surfaces for both wild type and mutant type Gram negative cells. Note that silica and anatase particles do not enter the cell, whereas alumina penetrates into the cell. Surface chemistry of cells and particles as well as particle size control penetration and toxicity.
Relative cell viability in the presence of titania without and with treatment by bovine liver catalaze. (BLC) scavenges ROS on the titania surface, which are toxic to cells; hence, cell viability is higher in the presence of BLC.
Relative toxicity of wild type and mutant type Gram negative and Gram positive cells. Note greater susceptibility of the latter to mineral toxicity. Cell wall structures are shown on the right.
EPS production induced by exposure of cells to toxic oxides.
Publications
- Xu, J., Campbell, J., Zhang, N., Hickey, W.J. & Sahai, N. (2012). Evolution of bacterial biofilms as armor against mineral toxicity. Astrobiology, 12: 785-798.
- Xu, J., Eggleston, C.M., Schoonen, M.A.A. & Sahai, N. (2012, in review). Formation mechanisms of reactive oxygen species in aqueous suspensions of oxide minerals: Implications for organic and cellular evolution on early Earth and extra-terrestrial worlds. Earth and Planetary Science Letters.
- Zhu, C., Xu, J., Hickey, W.J. & Sahai, N. (2012). Role of cell wall structure and extra-cellular polymeric substances (EPS) in shielding against specific mineral toxicity: Implications for cell surface evolution. Astrobiology.
-
PROJECT INVESTIGATORS:
-
PROJECT MEMBERS:
Jie Xu
Graduate Student
Chunxiao Zhu
Graduate Student
-
RELATED OBJECTIVES:
Objective 3.4
Origins of cellularity and protobiological systems
Objective 5.1
Environment-dependent, molecular evolution in microorganisms