2012 Annual Science Report
NASA Jet Propulsion Laboratory - Icy Worlds
Reporting | SEP 2011 – AUG 2012
Executive Summary
Our goal in the Astrobiology of the Icy Worlds Investigation is to advance our understanding of the role of ice in the broad context of astrobiology through a combined laboratory, numerical, analytical, and field investigations. Icy Worlds team will pursue this goal through four major investigations namely, the habitability, survivability, and detectability of life of icy worlds coupled with “Path to Flight” Technology demonstrations. We will also use a wealth of existing age-appropriate educational resources to convey concepts of astrobiology, spectroscopy, and remote sensing, and especially how these area interplay for learning new information; develop standards-based, hands-on activities to extend the application of these resources to the search for life on icy worlds. Our classification of “icy world” extends to planets, dwarf planets and small solar system bodies composed of a significant fraction (≥10%) of H2O under conditions of pressure and temperature that sustain water ice. By this definition, all objects of interest in the Solar System are smaller than Earth — though icy “ocean planets” (Leger et al., 2004) may exist around other stars. Glaciated regions of Mars and Earth resemble icy worlds, and these regions will serve as important analogues.
Icy worlds may harbor the greatest volume of habitable space in the Solar System. Beneath their icy surfaces, oceans and seas have been predicted for at least six worlds beyond Earth, yielding a total reservoir of some 30-40 times the volume of all the liquid water on Earth. Such worlds may be habitable if they host biogenic elements and mechanisms for maintaining chemical disequilibria. But the presence of life in these worlds is of little scientific value if our robotic and human explorers cannot discover the inhabitants. The floating shells of icy worlds serve as our only window into the interiors of these worlds – at least for near-future exploration. It is therefore critical to gain an understanding of what signs of life – or biosignatures – may survive on the surfaces or near surface zone of such worlds. The search for such signs requires instruments and techniques capable of detecting them. Advancing this capacity for discovery on other worlds can only be done through well-coordinated laboratory, field, and airborne campaigns of life here on Earth. It is through such campaigns, and an integrated approach to the habitability, survivability, and detectability of life on icy worlds, that we can advance astrobiology and further NASA’s capabilities for discovering life elsewhere while improving our understanding of life here on Earth.
Moving away from the Sun, water ice is ubiquitous on the Earth and beyond. A search for life linked to the search for water should naturally “follow the ice”. As mentioned above, such an approach leads to massive subsurface oceans, which evoke a sense of mystery not unrelated to that familiar to Earth’s global ocean. Can life emerge and thrive in a cold, lightless world beneath hundreds of kilometers of ice? And if so, do the icy shells hold clues to life in the subsurface? These questions are the primary motivation of our science investigations which are as follows:
o Habitability of Icy Worlds investigates the habitability of liquid water environments in icy worlds, with a focus on what processes may give rise to life, what processes may sustain life, and what processes may deliver that life to the surface.
o Survivability of Icy Worlds investigates the survivability of biological compounds under simulated icy world surface conditions, and compare the degradation products to abiotically synthesized compounds resulting from the radiation chemistry on icy worlds.
o Detectability of Icy Worlds investigates the detectability of life and biological materials on the surface of icy worlds, with a focus on spectroscopic techniques, and on spectral bands that are not in some way connected to photosynthesis.
o Our technology investigation, a Path to Flight for astrobiology, utilizes instrumentation built with non-NAI funding to carry out the science investigations discussed above. The search for life requires instruments and techniques that can detect biosignatures from orbit and in-situ under harsh conditions. Advancing this capacity is the focus of our Technology Investigation.
o Habitability of Icy Worlds investigation has three major objectives. Objective 1, Seafloor Processes, explores conditions that might be conducive to originating and supporting life in icy world interiors. To enable prediction testing by this and other teams pursuing related questions, we will define biomarker production and look for ground-truth in analog terrestrial systems. Objective 2, Ocean Processes, investigates the formation of prebiotic cell membranes under simulated deep-ocean conditions, computationally assesses the distribution of material and heat through the ocean and ice shell, and seeks to improve relevant chemical literature through a set of high-pressure equation-of-state experiments. Objective 3, Ice Shell Processes, investigates astrobiological aspects of ice shell evolution. Experimental work examines the creation of clathrate hydrates. Computational modeling of ice shell convection tracks the shuttling of these and other biorelevant materials between oceans and surfaces, and also examines the possibility of “sub-glacial” lakes as briny oases within the ice.
As part of Seafloor Processes where we have been testing through comprehensive laboratory studies the theory that life may have emerged ~4 billion years ago within porous and partly permeable submarine, moderate-temperature (≤100°C) alkaline hydrothermal springs on any wet, rocky planet or satellite in the Solar system, we continued to analyze hydrothermal simulation reactor effluents and investigated Fe, S, and C biogeochemical cycles. We considered relevance of a series of geochemical and electrochemical reactions at submarine alkaline vents as plausible harbingers of metabolism and life in the oceans of Icy Worlds.
We continued to carry out our membrane experiments with the demonstration of self-organizing dissipative structures which is to be expected in the far-from-equilibrium conditions obtaining at submarine alkaline hydrothermal springs (Mielke et al., 2011; Barge et al., 2012; McGlynn et al., 2012). Raman analyses of these hydrothermal chimneys using a newly fabricated rig confirmed that mackinawite (FeS) dominated the chimney walls at all temperatures, though crystal size reduced to nanocrystalline scales at 60°C and above (White et al., 2012).
Cover image by Laura M. Barge et al. of Icy Worlds Team. Chemical gardens containing iron, phosphate, and silicate form when an acidic FeCl2 solution is injected into an alkaline silicate/phosphate solution. Precipitation occurs immediately at the solution interface and continues as internal fluid pressure causes the membrane to rupture and re-precipitate repeatedly. This process results in self-assembling hollow inorganic membranes with various morphologies (bulbous structures, tubes, and plumes) that depend on experimental conditions. ESEM imaging and EDX elemental abundance analysis of precipitates show compositional differences within the membranes (ranging from iron-rich interior to silicate/phosphate-rich exterior), and during growth a membrane potential ranging from 150 to 200 mV was generated, indicating that electrochemical gradients are maintained across these semipermeable inorganic membranes. These experiments form a basis for understanding how chemical garden structures formed in natural systems such as alkaline hydrothermal vents which may have maintained similar electrochemical gradients, and whether this energy could be harnessed to fuel reactions within the membrane. For more information, see “Characterization of Iron-Phosphate-Silicate Chemical Garden Structures” by Laura M. Barge, Ivria J. Doloboff, Lauren M. White, Galen D. Stucky, Michael J. Russell, and Isik Kanik on pages 3714-3721.
As a part of the seafloor project, Co-I Vance, working with Lance Christensen, conducted a field trip and sampled serpentinizing fluids and gases from The Cedars, near Cazadero, CA, and Cabeço de Vide in central Portugal. Sampled gases show signatures of ethane and methane when analyzed using the Carbon Isotope Laser Spectrometer (CILS). These gases are known to exsolved from fluids at The Cedars, but have not yet been documented at the latter site. Detection using CILS is useful as an analogue to pending investigations by TLS on SAM on the Curiosity rover.
The intention for this work is to develop CILS as an instrument that can be used for rapidly characterizing and understanding serpentinizing systems and similar sites of interest as analogues to the origin of life on Earth, and on Mars and Europa. This instrumentation would also be of interest for deploying to Titan or the large outer planets in order to understand their composition and volatile processes. Dr. Vance shared his preliminary results with Portugese media, highlighting the importance in the context of understanding life’s possible origin in serpentinizing systems on Earth, Mars, and Europa.
We also continued to investigate mass and heat transport processes in icy world oceans.
Co-I Prof. Goodman and his student published results from the initial study of the 3-dimensional evolution of a plume in Europa’s ocean. A model run incorporating equations of state for MgSO4 has been performed and is being prepared for publication.
Co-I’s Vance and Brown implemented a spline-based method for generating self-consistent equations of state data for aqueous solutions. Application to existing sound velocity data from Vance’s thesis work has led to accurate representations of density, sound velocity, heat capacity, and related quantities over the full range of pressures, temperatures, and compositions relevant to icy world oceans. Initial considerations for Ganymede show that highly saline fluids are gravitationally stable under high-pressure ice layer at the base of Ganymede’s ocean, opening the possibility for water-rock interactions in large icy worlds. This work led to a joint, collaborative work with Drs. Sotin and Choukroun.
As part of our Survivability of icy Worlds investigation, we have continued to investigate the the abiotic chemistry of planetary ices irradiated with energetic particles electrons, ions, and ultraviolet (UV) photons and the chemistry of biomolecules exposed to similar conditions. The following summarizes our progress in this area.
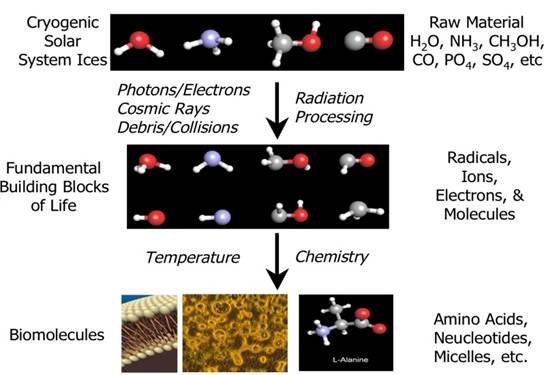
Radiation plays competing roles in the synthesis and destruction of compounds important to life. It can serve to synthesize organic compounds that serve as precursors to biomolecules, and it can break apart such compounds and destroy life itself. Chemistry on the surface of many icy worlds is driven by energetic ion, electron and photon bombardment.
In a new study, conducted by Gudipati and Yang and published in the Astrophysical Journal Letters (Gudipati and Yang, 2012), provides the first direct look at the organic chemistry that takes place on icy particles in the frigid reaches of our solar system, and in the even chillier places between stars. The organics looked at in the study are called polycyclic aromatic hydrocarbons, or PAHs for short. In this investigation, Murthy and his colleague simulated the environment of icy PAH molecules in the quiet cold of space, at temperatures as low as 5 K. First, they bombarded the particles with ultraviolet radiation similar to that from stars. Then, to determine the products of the chemical reaction, they used a type of laser system known as MALDI (for Matrix Assisted Laser Desorption and Ionization), which involves zapping the ice with both infrared and ultraviolet lasers. Their results revealed that the PAHs had transformed: they had incorporated hydrogen atoms into their structure and lost their circular, aromatic bonds, becoming more complex organics. According to the study, this is the type of change that would need to occur if the material were to eventually become amino acids and nucleotides — bits and pieces of protein and DNA, respectively.
Bacterial spores are one of the toughest and most durable forms of life on earth, and are consequently fascinating subjects of investigation for astrobiology. A new investigation into the spectral properties of bacterial spores in cryogenic ices and their viability under solar radiation has been started by co –Invesigator Paul Johnson and collaborator from the JPL Titan team, Robert Hodyss, along with JPL summer intern, Tucker Ely. This new investigation has been aimed to look into if bacterial spores embedded in near surface Europan ice can survive the Jovian radiation environment and if either the spores or their organic radiolysis products can be detected spectroscopically through remote sensing.
In addition, Co-Investigator Adrian Ponce started to look into viability assessment of microbes in simulated Europan radiation environment which focuses on the viability of spores and radiation resistant microbes in ices under radiation environment. He also studied Viability of Microbes Embedded in Kilimanjaro’s Glacial Ice as potential analog site for investigations of microbial survival and growth on icy worlds such as Mars, Europa and others. The results from this study are expected to further our understanding of microbial growth boundary conditions, habitability, and preservation of organics.
A growing body of literature reports investigations of high-pressure physiology of piezophiles and non-piezophiles, and their adaptations to extreme high-pressure environments. Recently, aerobic Bacillus spores have been shown to germinate as a result of being exposed to high hydrostatic pressures (50-800 MPa). To complete the picture, Ponce and his team has plans for high pressure microbiology investigations and look into the possibility of germination.
Detectability of life investigation has three major objectives: Detection of Life in the Laboratory, Detection of Life in the Field, and Detection of Life from Orbit. As part of the Detectability of Life investigation, Co-I Dalton and his team has been working on high-quality spectral characterization of anticipated, astrobiologically-significant Icy World surface components as well as identification of astrobiologically-relevant Icy World surface components from infrared spacecraft observations.
Most of our knowledge on Icy World’s surface composition comes from visible and infrared remote sensing. Spacecraft and telescopic instruments acquire spectra of the surface, and a model spectrum is constructed based on known spectra of the constituents, and the amounts of each constituent varied until a match is obtained. In the case of Europa, The Argadnel Regio observational spectra are considered to be a treasure trove of information about endogenic and exogenic (radiation-driven) chemistry, with unique insights to the interior composition. Dalton and his colleagues has utilized the Argadnel Regio reference spectra and developed models of electron and ion flux. They were able to show that their derived sulfuric acid hydrate abundances correlated well with electron energy and sulfur ion number density. They also found that some areas of the leading hemisphere and polar regions have received virtually no radiation, and may preserve original interior concentrations in their deposits. If microorganisms prove detectable at anticipated concentrations, these would be ideal places to search for them, by remote sensing or even with a lander.
In addition, Dalton and his colleagues have begun a major task of developing reference spectra for remote sensing for Europa which could also be useful for landing site selection by measuring simple inorganic and organic compounds, working our way up to more complex materials including actual microbial organisms.
As part of Investigation 3, Detectability of Life, Co-I Gordon Love and his team studied sediment cores from thaw lakes in Barrow, Alaska. Their objectives were to 1) investigate the production, preservation, and detection of chemotrophic lipid biological markers in Arctic thaw lakes as an analog for life detection under Icy Worlds conditions, 2) to generate historical, biomarker-based records of methane cycling and primary production in Arctic permafrost-bound lakes from sediment core analyses, and 3) to integrate biomarker records with parallel datasets regarding microbial ecology, water column chemistry and ice composition obtained by other team members. The results of these investigations and their significance for organic matter source and composition as well as methane cycling within the lakes were presented as a poster at the American Association of Petroleum Geochemists Student Expo and are the subject of a Master’s thesis of his graduate student Mark Williams.
Part of Investigation 3 also involves fieldwork on methane seeping lakes along the North Slope of Alaska conducted by Co-I Kevin Hand The science campaign by Co-I Hand made significant advances in limnology and biology, over the past year. During October of 2011 the Hand team continued analyses of the geochemistry and biology of the lake water, sediments and gases. They also deployed several new technologies for mapping and identification of seep areas. These prototypes – called Kite-Cam and Bubble-Cam provided overhead and direct views of the seep areas.
Figures show (a) Bubble-Cam being deployed, (b) a low-res image from Bubble-Cam, and© an image of the open-water seeps as captured by Kite-Cam from approximately 70 meters above the lake surface.
The (Field Instrumentation and) Path to Flight investigation’s purpose is to support science investigations in the field by enabling in-situ measurements of organics and biological material with field instrumentation (that have high potential for future flight instrumentation) and to provide new measurement capabilities that have been developed with the specific goal of life-detection. The instrument arsenal goes beyond the commercially available instrumentation and brings next generation imaging spectrometers, chromatographic, and sample extraction devices.
In an effort to tie together the in-situ instrumentation being developed as future flight instruments, Raman spectroscopy was employed for analysis of hydrothermal vent chimneys being generated in Investigation 1 by Ph.D candidate Lauren Spencer and Co-I M. Russell to better understand the mineralogy of these chimneys and specifically the effect of temperature and its effect on mineralogical species. The primary components being observed were greigite, mackinawite, and Fe(III) containing mackinawite – all of which catalyze the synthesis of organic compounds. In addition to the detection, characterization, and quantification of these mineral features, the results have fed back into instrument development efforts.
During year 4, our effort was placed on analyzing the data collected from a series of successful ocean/ocean subsurface deployments of the deep biosphere borehole instrument (DEBI-T) that was collaboratively built by Photon Systems, JPL, Lamont-Doherty Columbia Unviersity, and USC under funding from the NAI-Icy Worlds, NSF, C-DEBI, and Sloan foundation. The instrument is a novel deep ocean, deep UV native fluorescence instrument for subsurface in-situ detection of organics and microbes living on borehole walls and/or in the water column. The development of the instrument was spurred by results of label-free microbial detection that was demonstrated in part, in year 1 and 2 of the Icy Worlds NAI (Bhartia et al., 2010).
As a part of Investigation 4, Principal Investigator I. Kanik and his group continued with Purdue University to collaboratively developed Portable Mini 10 Mass Spectrometer (MS) system (Sokol et al., 2011; Soparawalla et al., 2012)) and utilized it in the Field, developed and incorporated robotic analytical chemistry laboratory also known as RCAL system onto a rover at JPL’s Mars yard for field demonstration.
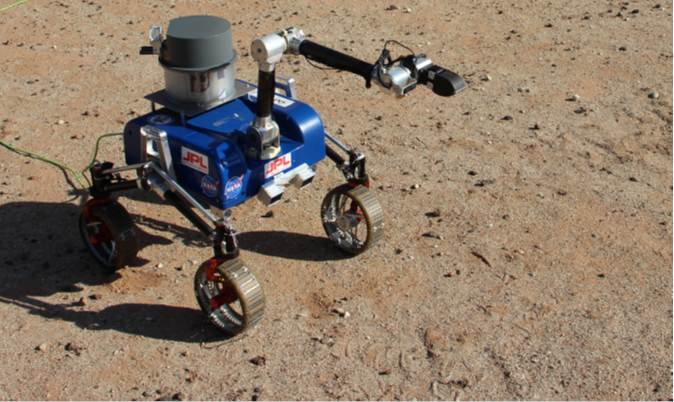
RCAL (robotic chemistry lab) had been mounted on the SRR-2K rover and all power and controls now come from the rover and successfully collected soil samples and analyze it. Earlier trials had identified shortcomings in some of the hardware and many actuators have now been replaced by encoded motors to give more reliable and precise control, coupled with appropriate changes to the operations software.
As a part of Investigation 4, Path to Flight, we fully designed a low-cost flyby concept, called LIFE, for a sample return mission to Enceladus, a body with high astrobiological potential. The LIFE mission concept is envisioned to orbit Saturn (both to achieve lower sampling speeds approaching 2 km/s which would enable more gentle sample collection than Stardust and to make possible multiple flybys of Enceladus), to sample Enceladus’ plume, the E ring of Saturn and the Titan upper atmosphere. (Tsou et al., 2012).
References:
Bhartia, R., Salas, E. C.; Hug, W. F.; et al., Label-Free Bacterial Imaging with Deep-UV-Laser-Induced Native Fluorescence, (2010) APPLIED AND ENVIRONMENTAL MICROBIOLOGY, 76 Issue: 21 Pages: 7231-7237.
Publications
-
Barge, L. M., Doloboff, I. J., Russell, M. J., VanderVelde, D., White, L. M., Stucky, G. D., … Kanik, I. (2014). Pyrophosphate synthesis in iron mineral films and membranes simulating prebiotic submarine hydrothermal precipitates. Geochimica et Cosmochimica Acta, 128, 1–12. doi:10.1016/j.gca.2013.12.006
-
Barge, L. M., Doloboff, I. J., White, L. M., Stucky, G. D., Russell, M. J., & Kanik, I. (2012). Characterization of Iron–Phosphate–Silicate Chemical Garden Structures. Langmuir, 28(8), 3714–3721. doi:10.1021/la203727g
-
Barnett, I. L., Lignell, A., & Gudipati, M. S. (2012). SURVIVAL DEPTH OF ORGANICS IN ICES UNDER LOW-ENERGY ELECTRON RADIATION (⩽2 keV). The Astrophysical Journal, 747(1), 13. doi:10.1088/0004-637x/747/1/13
-
Bhartia, R., Salas, E. C., Hug, W. F., Reid, R. D., Lane, A. L., Edwards, K. J., & Nealson, K. H. (2010). Label-Free Bacterial Imaging with Deep-UV-Laser-Induced Native Fluorescence. Applied and Environmental Microbiology, 76(21), 7231–7237. doi:10.1128/aem.00943-10
-
Boduch, P., Da Silveira, E. F., Domaracka, A., Gomis, O., Lv, X. Y., Palumbo, M. E., … Strazzulla, G. (2011). Production of Oxidants by Ion Bombardment of Icy Moons in the Outer Solar System. Advances in Astronomy, 2011, 1–10. doi:10.1155/2011/327641
-
Boduch, P., Domaracka, A., Fulvio, D., Langlinay, T., Lv, X. Y., Palumbo, M. E., … Strazzulla, G. (2012). Chemistry induced by energetic ions in water ice mixed with molecular nitrogen and oxygen. A&A, 544, A30. doi:10.1051/0004-6361/201219365
-
Brad Dalton, J., Cruikshank, D. P., & Clark, R. N. (2012). Compositional analysis of Hyperion with the Cassini Visual and Infrared Mapping Spectrometer. Icarus, 220(2), 752–776. doi:10.1016/j.icarus.2012.05.003
-
Branscomb, E., & Russell, M. J. (2013). Turnstiles and bifurcators: The disequilibrium converting engines that put metabolism on the road. Biochimica et Biophysica Acta (BBA) – Bioenergetics, 1827(2), 62–78. doi:10.1016/j.bbabio.2012.10.003
-
Cooper, P. D. (2012). Oxygen in the Outer Solar System. Journal of Chemical Education, 89(2), 181–182. doi:10.1021/ed200013q
-
Dalle Ore, C. M., Fulchignoni, M., Cruikshank, D. P., Barucci, M. A., Brunetto, R., Campins, H., … Strazzulla, G. (2011). Organic materials in planetary and protoplanetary systems: nature or nurture?. A&A, 533, A98. doi:10.1051/0004-6361/201116599
-
Dalton, J. B., & Pitman, K. M. (2012). Low temperature optical constants of some hydrated sulfates relevant to planetary surfaces. Journal of Geophysical Research: Planets, 117(E9), n/a–n/a. doi:10.1029/2011je004036
-
Dalton, J. B., Cassidy, T., Paranicas, C., Shirley, J. H., Prockter, L. M., & Kamp, L. W. (2013). Exogenic controls on sulfuric acid hydrate production at the surface of Europa. Planetary and Space Science, 77, 45–63. doi:10.1016/j.pss.2012.05.013
-
Dalton, J. B., Shirley, J. H., & Kamp, L. W. (2012). Europa’s icy bright plains and dark linea: Exogenic and endogenic contributions to composition and surface properties. Journal of Geophysical Research: Planets, 117(E3), n/a–n/a. doi:10.1029/2011je003909
-
Do, N. H., & Cooper, P. D. (2013). Formation and Reaction of Oxidants in Water Ice Produced from the Deposition of RF-Discharged Rare Gas and Water Mixtures. The Journal of Physical Chemistry A, 117(1), 153–159. doi:10.1021/jp3090556
-
Goodman, J. C., & Lenferink, E. (2012). Numerical simulations of marine hydrothermal plumes for Europa and other icy worlds. Icarus, 221(2), 970–983. doi:10.1016/j.icarus.2012.08.027
-
Gudipati, M. S., & Yang, R. (2012). IN-SITU PROBING OF RADIATION-INDUCED PROCESSING OF ORGANICS IN ASTROPHYSICAL ICE ANALOGS—NOVEL LASER DESORPTION LASER IONIZATION TIME-OF-FLIGHT MASS SPECTROSCOPIC STUDIES. The Astrophysical Journal, 756(1), L24. doi:10.1088/2041-8205/756/1/l24
-
Hand, K. P., & Carlson, R. W. (2012). Laboratory spectroscopic analyses of electron irradiated alkanes and alkenes in solar system ices. Journal of Geophysical Research: Planets, 117(E3), n/a–n/a. doi:10.1029/2011je003888
-
Hodyss, R., Howard, H. R., Johnson, P. V., Goguen, J. D., & Kanik, I. (2011). Formation of radical species in photolyzed CH4:N2 ices. Icarus, 214(2), 748–753. doi:10.1016/j.icarus.2011.05.023
-
Ivanov, M. A., Prockter, L. M., & Dalton, B. (2011). Landforms of Europa and selection of landing sites. Advances in Space Research, 48(4), 661–677. doi:10.1016/j.asr.2011.05.016
-
Johnson, P. V., Hodyss, R., Chernow, V. F., Lipscomb, D. M., & Goguen, J. D. (2012). Ultraviolet photolysis of amino acids on the surface of icy Solar System bodies. Icarus, 221(2), 800–805. doi:10.1016/j.icarus.2012.09.005
-
Korablev, O., Gerasimov, M., Brad Dalton, J., Hand, K., Lebreton, J-P., & Webster, C. (2011). Methods and measurements to assess physical and geochemical conditions at the surface of Europa. Advances in Space Research, 48(4), 702–717. doi:10.1016/j.asr.2010.12.010
-
Lazcano, A., & Hand, K. P. (2012). Astrobiology: Frontier or fiction. Nature, 488(7410), 160–161. doi:10.1038/488160a
-
Lv, X. Y., De Barros, A. L. F., Boduch, P., Bordalo, V., Da Silveira, E. F., Domaracka, A., … Strazzulla, G. (2012). Implantation of multiply charged carbon ions in water ice. A&A, 546, A81. doi:10.1051/0004-6361/201219886
-
McGlynn, S. E., Kanik, I., & Russell, M. J. (2012). Peptide and RNA contributions to iron-sulphur chemical gardens as life’s first inorganic compartments, catalysts, capacitors and condensers. Philosophical Transactions of the Royal Society A: Mathematical, Physical and Engineering Sciences, 370(1969), 3007–3022. doi:10.1098/rsta.2011.0211
-
Mielke, R. E., Robinson, K. J., White, L. M., McGlynn, S. E., McEachern, K., Bhartia, R., … Russell, M. J. (2011). Iron-Sulfide-Bearing Chimneys as Potential Catalytic Energy Traps at Life’s Emergence. Astrobiology, 11(10), 933–950. doi:10.1089/ast.2011.0667
-
Murray, A. E., Kenig, F., Fritsen, C. H., McKay, C. P., Cawley, K. M., Edwards, R., … Doran, P. T. (2012). Microbial life at -13 C in the brine of an ice-sealed Antarctic lake. Proceedings of the National Academy of Sciences, 109(50), 20626–20631. doi:10.1073/pnas.1208607109
-
Nitschke, W., & Russell, M. J. (2011). Redox bifurcations: Mechanisms and importance to life now, and at its origin. BioEssays, 34(2), 106–109. doi:10.1002/bies.201100134
-
Nitschke, W., & Russell, M. J. (2013). Beating the acetyl coenzyme A-pathway to the origin of life. Philosophical Transactions of the Royal Society B: Biological Sciences, 368(1622), 20120258–20120258. doi:10.1098/rstb.2012.0258
-
Nitschke, W., McGlynn, S. E., Milner-White, E. J., & Russell, M. J. (2013). On the antiquity of metalloenzymes and their substrates in bioenergetics. Biochimica et Biophysica Acta (BBA) – Bioenergetics, 1827(8-9), 871–881. doi:10.1016/j.bbabio.2013.02.008
-
Noell, A. C., Greenwood, A. R., Lee, C. M., & Ponce, A. (2013). High-density, homogeneous endospore monolayer deposition on test surfaces. Journal of Microbiological Methods, 94(3), 245–248. doi:10.1016/j.mimet.2013.05.003
-
Pearce, M. P., Bussemaker, M. J., Cooper, P. D., Lapere, K. M., Wild, D. A., & McKinley, A. J. (2012). Formation of methanol from methane and water in an electrical discharge. Physical Chemistry Chemical Physics, 14(10), 3444. doi:10.1039/c2cp22135g
-
Russell, M. J., Nitschke, W., & Branscomb, E. (2013). The inevitable journey to being. Philosophical Transactions of the Royal Society B: Biological Sciences, 368(1622), 20120254–20120254. doi:10.1098/rstb.2012.0254
-
Savin, D. W., Brickhouse, N. S., Cowan, J. J., Drake, R. P., Federman, S. R., Ferland, G. J., … Zweibel, E. G. (2012). The impact of recent advances in laboratory astrophysics on our understanding of the cosmos. Reports on Progress in Physics, 75(3), 036901. doi:10.1088/0034-4885/75/3/036901
-
Schoepp-Cothenet, B., Van Lis, R., Atteia, A., Baymann, F., Capowiez, L., Ducluzeau, A-L., … Nitschke, W. (2013). On the universal core of bioenergetics. Biochimica et Biophysica Acta (BBA) – Bioenergetics, 1827(2), 79–93. doi:10.1016/j.bbabio.2012.09.005
-
Schoepp-Cothenet, B., Van Lis, R., Philippot, P., Magalon, A., Russell, M. J., & Nitschke, W. (2012). The ineluctable requirement for the trans-iron elements molybdenum and/or tungsten in the origin of life. Scientific Reports, 2. doi:10.1038/srep00263
-
Shibuya, T., Tahata, M., Ueno, Y., Komiya, T., Takai, K., Yoshida, N., … Russell, M. J. (2013). Decrease of seawater CO2 concentration in the Late Archean: An implication from 2.6Ga seafloor hydrothermal alteration. Precambrian Research, 236, 59–64. doi:10.1016/j.precamres.2013.07.010
-
Simoncini, E., Russell, M. J., & Kleidon, A. (2011). Modeling Free Energy Availability from Hadean Hydrothermal Systems to the First Metabolism. Orig Life Evol Biosph, 41(6), 529–532. doi:10.1007/s11084-011-9251-4
-
Sokol, E., Noll, R. J., Cooks, R. G., Beegle, L. W., Kim, H. I., & Kanik, I. (2011). Miniature mass spectrometer equipped with electrospray and desorption electrospray ionization for direct analysis of organics from solids and solutions. International Journal of Mass Spectrometry, 306(2-3), 187–195. doi:10.1016/j.ijms.2010.10.019
-
Tsou, P., Brownlee, D. E., McKay, C. P., Anbar, A. D., Yano, H., Altwegg, K., … Kanik, I. (2012). LIFE: Life Investigation For Enceladus A Sample Return Mission Concept in Search for Evidence of Life. Astrobiology, 12(8), 730–742. doi:10.1089/ast.2011.0813
-
Vance, S., & Michael Brown, J. (2013). Thermodynamic properties of aqueous MgSO4 to 800MPa at temperatures from −20 to 100°C and concentrations to 2.5molkg−1 from sound speeds, with applications to icy world oceans. Geochimica et Cosmochimica Acta, 110, 176–189. doi:10.1016/j.gca.2013.01.040
-
Wilson, J. P., Grotzinger, J. P., Fischer, W. W., Hand, K. P., Jensen, S., Knoll, A. H., … Tice, M. M. (2012). DEEP-WATER INCISED VALLEY DEPOSITS AT THE EDIACARAN-CAMBRIAN BOUNDARY IN SOUTHERN NAMIBIA CONTAIN ABUNDANT TREPTICHNUS PEDUM. PALAIOS, 27(4), 252–273. doi:10.2110/palo.2011.p11-036r
- Beaty, S.M., Connon, S.A. & et al. (2012, In Preparation). Microbial Diversity of Kilimanjaro’s Glacial Ice. Environmental Microbiology.
- Bhartia, R., Hug, W.F., Fries, M., Reid, R., White, L. & Salas, E. (In Preparation). Sample Alteration and Solutions for Planetary Science. Astrobiology.
- Gudipati, M.S. & Cooper, P.D. Chemistry in Water Ices: From Fundamentals to Planetary Applications; The Science of Solar System Ices. In: Gudipati, M.S. & Castillo, J. (Eds.). New York: Springer.
- Mastrapa, R.M.E., Grundy, W.M. & Gudipati, M.S. (In Press). Amorphous and Crystalline H2O-Ice;; The Science of Solar System Ices. In: Gudipati, M.S. & Castillo-Rogez, J. (Eds.). New York: Springer.
- Noell, A.C., Abbey, W.J. & et al. (2012, Submitted). Age of Glacial Dust Layers and Soils at Kilimanjaro’s Northern Ice Field. Journal of Geophysical Research: Biogeosciences.
- Priscu, J.C. & Hand, K.P. (2012). Microbial Habitability of Icy Worlds. Microbe, 7(4): 167-172.
- White, L., Bhartia, R., Stucky, G., Kanik, I. & Russell, M. (2012, Submitted). Determining Catalytic Iron-sulfide Species in Ancient Alkaline Hydrothermal Vent Systems. Earth and Planetary Science.
- White, L.M., Bhartia, R., Stucky, G.D., Kanik, I. & Russell, M.J. (In Review). Characterizing compositional variations in catalytic iron-sulfide species in ancient alkaline hydrothermal vent systems. Earth and Planetary Science Letters.