2012 Annual Science Report
NASA Jet Propulsion Laboratory - Icy Worlds
Reporting | SEP 2011 – AUG 2012
Detectability of Life
Project Summary
Detectability of Life investigates the detectability of chemical and biological signatures on the surface of icy worlds, with a focus on spectroscopic techniques, and on spectral bands that are not in some way connected to photosynthesis.Detectability of life investigation has three major objectives: Detection of Life in the Laboratory, Detection of Life in the Field, and Detection of Life from Orbit.
Project Progress
Overview
Investigation, led by Co-I Dalton (JPL) with assistance from Co-Is Hand (JPL) and Ponce (JPL), and NASA Postdoctoral Program (NPP) fellow C. Jamieson and conducted at the JPL Planetary Ice Characterization Laboratory (PICL), focused on two main efforts: (1) High-quality spectral characterization of anticipated, astrobiologically-significant Icy World surface components and (2) Identification of astrobiologically-relevant Icy World surface components from infrared spacecraft observations. This prepares us for future operations addressing detectability of microorganisms – we are working up to the capability of conducting the same operations but with microorganisms as the surface component.
Galileo Near-Infrared Mapping Spectrometer (NIMS) observation of Argadnel Regio, Europa, overlain upon Galileo Solid-State Imager (SSI) photo. Each pixel of the false-color NIMS observation has a spectrum, extending into the page. The observation has been processed so that areas containing more water ice, such as the background ridged plains, appear bluish-purple, while areas containing less water ice and more hydrated compounds, primarily hydrated sulfate salts in this case, appear more reddish. These are prevalent where the icy crust has fractured and pulled apart, allowing endogenic material from the interior to fill in. This material is subsequently exposed to charged particle radiation in the form of electrons and ions striking the surface, driving chemistry in the surface deposits.
Galileo NIMS spectral average from a dark deposit on Europa (black line with error bars) and the best-fit model result using all cryogenic laboratory reference spectra measured at 77, 100 or 120 Kelvins (blue line and circles). Abundances of constituent materials are given as weight percent.
The bulk of our knowledge of Icy World surface composition comes from visible and infrared remote sensing. Spacecraft and telescopic instruments acquire spectra of the surface, either individually (point spectrometer) or in an array of geometrically registered spectra known as an image cube (imaging spectrometer). An image cube is like an image in which each pixel has a third, spectral dimension. Analysis of visible-infrared observations proceeds via modeling, whereby a model spectrum is constructed based on known spectra of the constituents, and the amounts of each constituent varied until a match is obtained. This type of analysis cannot be performed unless reference spectra of the surface constituents – measured at the surface temperature – have been acquired under controlled conditions and made available for modelers.
Reference Spectra and Modeling of Surface Deposits on Europa
The Argadnel Regio, Europa, observation shown in Figure 3-1 is a treasure trove of information about endogenic and exogenic (radiation-driven) chemistry, with unique insights to the interior composition. It contains several different geologic terrain types, which we assume formed through different processes. There are enough pixels in many geologic units to form statistically significant spectral averages using spectra extracted from a single unit. The observation straddles the meridian between the leading hemisphere (right) and the trailing hemisphere (left).
Because Europa’s orbital period (~3.5 days) is much longer than Jupiter’s rotation period of about ten hours, charged particles (electrons and H, S, O, Na, and other ions) trapped in the Jovian magnetosphere continually impinge upon Europa’s surface, driving radiolytic chemistry and producing hydrated sulfuric acid (H2SO4•nH2O) from surface materials. The peak of the distribution, to first order, lies near the apex of the trailing hemisphere and falls off with a cosine distribution as you approach the leading hemisphere. We have demonstrated (Shirley et al., 2010) a gradient in sulfuric acid abundance across the Argadnel Regio observation, with acid abundance increasing toward the trailing hemisphere. In 2011, we extended our analysis to additional, regionally-distributed observations to see if this was a global trend (Dalton et al., 2012b).
Europa global image mosaic, viewed from the anti-Jovian hemisphere direction, and overlain with a model of electron energy in MeV / cm2-s. Additional overlays (magenta-purple) denote NIMS observations analyzed for H2SO4 hydrate content: G1ENNHILAT01, 14ENSUCOMP01 (Argadnel Regio), 14ENSUCOMP03, and 17ENSUCOMP02 (near South Pole). Hydrate content was derived using laboratory reference spectra of water ice, sulfate brines, hydrated salts, and H2SO4 hydrate measured at 77, 100 and 120K.
H2SO4 hydrate abundance versus electron energy. Each point represents a modeled spectral average from the NIMS observations in Figure 3-3 (with the addition of observation 15ENSUCOMP01, not shown, near the leading hemisphere apex). Note the cluster of points derived from various terrains in Argadnel Regio. The data reveal a distinct correlative trend in H2SO4 abundance from high to low electron energy.
Teaming up with collaborators C. Paranicas and T. Cassidy, who have developed models of electron and ion flux that are more advanced than the simple inverse cosine falloff described earlier, we were able to show that our derived sulfuric acid hydrate abundances correlated well with electron energy and sulfur ion number density, but not as well with ion energy (Dalton et al., 2012c). A valuable result from this work is that while most of Europa’s surface bears evidence of an “overprinting” of sulfuric acid hydrate that is correlated to radiation dosage, certain exposures have lower H2SO4 hydrate abundances, suggesting that they have not yet reached the equilibrium state where creation and destruction of H2SO4 hydrate match and are therefore geologically young. We also found that some areas of the leading hemisphere and polar regions have received virtually no radiation, and may preserve original interior concentrations in their deposits. If microorganisms prove detectable at anticipated concentrations, these would be ideal places to search for them, by remote sensing or even with a lander.
Strategies for Landing Site Selection on Europa
We were called upon by both the Russian International Space Institute (IKI) and the NASA Outer Planets Assessment Group (OPAG) Europa Lander Forum to advise them on lander site selection for Europa. Most of the surface of Europa is extremely rough, and affords little opportunity for landing (Ivanov et al., 2011). Furthermore, surface materials near the trailing hemisphere apex are highly processed by incident radiation, and likely bear little resemblance to the interior composition. There are a few areas that have been imaged at high resolution where it appears that dark material from the interior has flowed onto the surface and cooled to form a relatively smooth surface. However, none of these are in the low-radiation zones we have identified on the leading hemisphere. Hence, we recommend that additional observations (imaging, and imaging spectroscopy) be acquired for the leading hemisphere to search for such a location, prior to delivery of a lander. This may be done on the same mission that carries the lander, or a prior mission.
Reference Spectra for Remote Sensing
Analysis of visible to infrared spectra and spectral images to retrieve surface abundances requires availability of reference spectra for the sought constituents. We have completed development and checkout of the Planetary Ice Characterization Facility (PICL) and have begun measuring simple inorganic and organic compounds, working our way up to more complex materials including actual microbial organisms.
Visible to infrared spectra of hexahydrite, MgSO4•6H2O. Believed to be a major constituent of Europa’s ocean and surface deposits, hexahydrite is a critical component of any model of Europa’ surface composition. Yet, these are the first published spectra of hexahydrite at 90-300 Kelvins that cover the full wavelength range of the Galileo and Cassini imaging spectrometers.
Visible to infrared spectra of methanol ice. Shown as an example of a simple organic molecule, these are the first cryogenic spectra of methanol ice taken in reflectance, at icy satellite temperatures, over the full wavelength range of the Galileo and Cassini imaging spectrometers. These spectra will enable detection of organic molecules, if present, by more accurate spectral models than ever before possible.
We began with the hydrated sulfate salts, such as hexahydrite (Figure 3-6) due to their prevalence in spectral and chemical models of Europa’s surface and interior. A composite model of surface deposits on Europa will require access to such spectra. Our comprehensive study includes six gran sizes for each compound to facilitate derivation of optical constants (real and imaginary indices of refraction; Dalton and Pitman, 2012). Availability of optical constants enables development of more advanced spectral models based on radiative transfer (Hapke, 1981). We intend to measure the reflectance of each compound at 50, 75 and 100 Kelvin, and at 25 Kelvin intervals, up to either 300K or the freezing point of the compound.
The PICL can measure spectra of solid minerals, or of ices introduced as liquids or deposited from the vapor phase. The methanol ice spectra in Figure 3-6 are from a vapor-deposited sample. In truth, the methanol ice does not exhibit a great deal of temperature sensitivity, at least not as compared with hydrated molecules like hexahydrite. Still, this cannot be known until the spectra have been measured, so this is not a waste of effort. Our next step is to examine more complex biological materials, including microorganisms. Prior work (Dalton et al., 2003) suggests that the microorganisms will continue to appear different at cryogenic temperatures than at room temperature. Direct detection of microorganisms by remote sensing, then, will require laboratory reference spectra of the type we plan to acquire next year.
Composition of Hyperion with Cassini VIMS
We applied our spectral remote sensing approach to Cassini Visual and Infrared Mapping Spectrometer (VIMS) observations of Saturn’s irregular satellite, Hyperion. Hyperion travels in a retrograde orbit, and tumbles chaotically as well. Long surmised to be a captured body, Hyperion’s origin has remained a mystery. Using VIMS observations (but no Cassini Data Analysis funds) we determined distributions of several molecules on Hyperion (Dalton et al., 2012a). Figure 3-7 is a Cassini image of Hyperion with two VIMS observations overlain in false color. Bluish tones represent water ice, while reddish tones represent carbon dioxide ice. The large amounts of pink/purple indicate both of these ices mixed together.
Hyperion imaged by Cassini Imaging Science Subsystem (ISS). Overlain are two moderate-resolution VIMS observations in false color. Blue =H2O ice; Red=CO2 ice; Yellow=adsorbed H2. Prevalent pink/purple indicates H2O and CO2 together.
High-resolution ISS image of Hyperion. Subcircular depressions reveal evidence of sublimation degradation, mass wasting, and redeposition of bright ice along ridges. Dark lag deposits contain accumulated and likely chemically processed contaminants.
Close examination of Figures 3-7 and 3-8 reveals that what first appear to be craters do not follow the typical model: they are not bowl-shaped, the walls show evidence of modification, likely by sublimation degradation and mass wasting, and there are often dark deposits, not shadows, at the bottom. These lag deposits are probably formed by solar heating of the dark material driving off the surrounding ice, leading to mass wasting and the concentration of contaminants in the dark deposits. We actually found very little pure, pristine ice: there was always some small amount of other material required to match the spectra. The yellow color associated with the dark deposits we have assigned to adsorbed H2, likely produced by photolysis of H2O ice. However we should mention that organic molecules bearing carbon-nitrogen triple bonds also have absorption features in the same portion of the infrared that was active in these deposits. Figure 3-9 is color-coded the same as Figure 3-7, with blue for H2O, red for CO2, pink indicating both, and yellow denoting accumulations of H2. In addition to these, we found evidence for both aliphatic (~3.9 µm) and aromatic (~3.8 µm) molecules in spectra of the background water/CO2 ice, distributed throughout the surface. In light of the presence of such contaminants throughout the ice of Hyperion, not just in the lag deposits, we suggest that it is either a captured comet or Kuiper Belt Object (KBO).
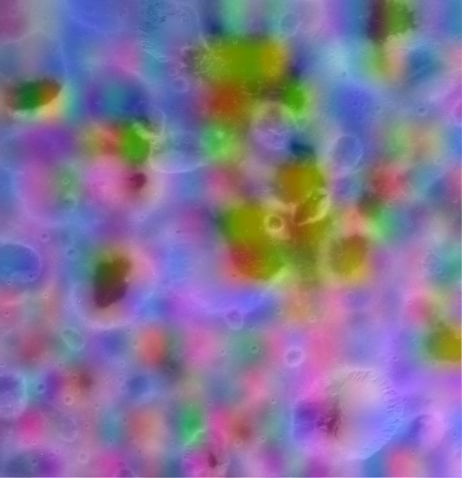
High-resolution ISS image of Hyperion, overlain with false colors from VIMS. Blue =H2O ice; Red=CO2 ice; Yellow=adsorbed H2. Prevalent pink/purple indicates H2O and CO2 together.
As part of Investigation 3, Detectability of Life, Co-I Gordon Love and his team studied sediment cores from thaw lakes in Barrow, Alaska. This science field campaign by Co-I Gordon made significant advances in geochemistry over the past year as reported below.
Their objectives were to 1) investigate the production, preservation, and detection of chemotrophic lipid biological markers in Arctic thaw lakes as an analog for life detection under Icy Worlds conditions, 2) to generate historical, biomarker-based records of methane cycling and primary production in Arctic permafrost-bound lakes from sediment core analyses, and 3) to integrate biomarker records with parallel datasets regarding microbial ecology, water column chemistry and ice composition obtained by other team members.
Progress
Extractable saturated hydrocarbons, silylated total lipid extracts as well as catalytic hydropyrolysis (HyPy) products from whole sediments were subjected to GC-MS and MRM-GC-MS analyses. The results of these analyses and their significance for organic matter source and composition (Table 3-1), and methane cycling within the lakes were presented as a poster at the American Association of Petroleum Geochemists Student Expo (Williams, et al., 2012) and are the subject of a Masters thesis (Mark Williams, UCR, 2012).
Biomarker interpretation table. Table of key lipid biomarkers and biomarker ratios and how they are interpreted.
Results
i) Sedimentary organic matter concentration and composition varies widely among lakes (Table 3-2), with Lake Q yielding the lowest average total organic carbon (TOC) concentration, and Sukok lake center/seep-distal site yielding the highest average TOC.
ii) Organic matter sources inferred from sedimentary organic matter composition (Table 3-1 and Table 3-2) indicate that although all lakes have some contribution from terrestrial plants, there is also strong evidence for abundant in situ microbial primary production (Figure 3-10), particularly by green algae and cyanobacteria (Figure 3-11). This is a major finding of our study. We conservatively estimate that >50% of sedimentary organic matter in some lakes may come from present-day/recent in situ microbial production. This has major implications for the composition and abundance of substrates for future sedimentary methanogenesis, and contradicts some previous published work implying that the bulk of organic matter in the lakes derives from Pleistocene-age and modern terrestrial plant input.
Selected features of lake sediment lipid biomarkers. Average TOC (Total Organic Carbon) of sediments analyzed; Sterane/Hopane ratio calculated from HyPy products; 3-MeHI, 3-methylhopane abundance as a percentage of the C30 αβ hopane in HyPy products; 2-MeHI, 2-methylhopane abundance as a percentage of the C30 αβ hopane in HyPy products; Arch/n-C31, archaeol as a percentage of the C31 n-alkane in BSTFA-derivatized total lipid extracts.
Distributions of linear hydrocarbons in Total Ion Chromatograms from North Walakpa (NW) and Sukok Seep (SS) hydropyrolysis products.
Abundance of 2-methylhopanes, most likely derived from cyanobacteria, as (left) a percentage of C30 αβ hopane with depth, and (right) relative to Total Organic Carbon (TOC). 2-Methylhopane Index (2-MeHI) varies with depth in the sediments and shows a positive linear relationship with total organic carbon content with different slopes for Sukok and North Walakpa. The 2-methylhopane index is calculated as a percentage with the C30 αβ hopane (C31 2-MeH/ (C30 αβH + C31 2-MeH)*100).
iii) Low but detectable amounts of archaeol (up to 1 ppm of total sedimentary organic carbon), a compound produced by archaea including methanogens, are present in all samples (Figure 3-12). Archaeol exhibits a positive relationship with TOC. This data will be compared with porewater methane concentrations as an additional check on whether it reflects greater methanogen abundance associated with greater substrate availability. Archaeol abundance was highest in lake sediment cores where active sub-surface methnogenesis is thought to be occurring (S3 and NW) and lowest in Sukok Seep (SS) core where methane radiocarbon dates have identified an older and deeper source of coalbed methane.
Distribution of archaeol, a compound produced by (methanogenic/methanotrophic) archaea in Total Ion Chromatograms from Sukok Seep and North Walakpa silylated total lipid extracts. Insets magnify portion of the chromatogram in which archaeol elutes.
iv) Lipid biomarkers known to derive from marine anaerobic methane-oxidizing archaea (pentamethylicosane, crocetane, biphytane) were below detection limits in all samples analysed..
iv) Low but detectable amounts of lipid compounds produced by certain groups of aerobic methanotrophic bacteria (3-methylhopanes, Table 1, Figure 3-13) were present in all lakes. Although type I methanotrophs are expected to be the dominant clade in cold, freshwater systems, these compounds likely underestimate total methanotroph abundance because other methanotrophic types do not produce 3-methylhopanoid biomarkers. 3-methylhopanes abundances do not correlate with TOC content of the underlyting sediments, perhaps reflecting the presence of geogenic methane in lower-TOC sediments, or perhaps the short water column residence time of methane bubbles rising rapidly through the sediments as opposed to diffuse methane that lingers in sediment pore waters.
3-methylhopanes most likely derived from aerobic methanotrophic bacteria as (left) a function of depth in the sediments in (left) freely extractable hydrocarbons and (right) hydropyrolysis products. 3-methylhopanes show no correlation with total organic carbon. The 3-methylhopane index is calculated as a percentage with the C30 αβ hopane (C31 3-MeH/ (C30 αβH + C31 3-MeH)*100).
Plans for future work
In the final year of the project:
i) Compound-specific carbon isotope analyses will be conducted on free hydrocarbons and hydropyrolysis products to test for significant 13C-depletion of key compounds such as hopanes (thus confirming a contribution from methane-oxidizing microbes).
ii) Radiocarbon dating of organic matter in the cores will be used to construct age models for each lake, which will then be used to aid in paleoclimatic interpretation of the lipid biomarker records.
iii) A longer sediment core will be collected from North Walakpa in Fall 2012 for the construction of a higher-resolution lipid biomarker record in conjunction with complementary pore water geochemistry.
iv) We integrate biomarker lipid results with findings from our collaborators at DRI and Montana State on the microbial ecology of the lake water and underlying sediments.
v) A manuscript reporting general lipid biomarker results will be submitted to Organic Geochemistry in early 2013, and a manuscript reviewing the utility of lipid biomarkers in the astrobiology of icy worlds will be contributed to the NAI team special issue of Astrobiology.
Part of Investigation 3 also involves fieldwork on methane seeping lakes along the North Slope of Alaska. They continued analyses of the geochemistry and biology of the lake water, sediments and gases. The science campaign by Co-I Hand made significant advances in limnology and biology, over the past year as reported below. During October of 2011 they also deployed several new technologies for mapping and identification of seep areas. These prototypes – called Kite-Cam and Bubble-Cam provided overhead and direct views of the seep areas.
Limnology
One of the major purposes of the October 2011 field campaign program was to collect:
o Physical and chemical profiles from selected lakes
o Samples for methane oxidation
o Bacterial productivity measurements as a function of temperature
o In addition we completed analyzing bacteria within lake ice cores collected during the April 2010 campaign and initiated next generation sequencing of samples collected during past campaigns.
Location, depth and ice thickness for the October 2011 study lakes.
Vertical profiles of physical and chemical factors show clear differences among the lakes (Fig. 3-14). Water column temperatures ranged from 0.12 to 2.3 oC and, in concert with the conductivity data, revealed relatively stable water columns, with denser water near the bottom. The exception to this was the Sukok Seep site where temperature and conductivity were uniform with depth, presumable as the result of turbulent mixing by rising methane bubbles. The lakes were typically well oxygenated to the bottom except for the lake North of Walapka, which showed a distinct decrease in oxygen with depth reaching 0.5 mg L-1 just off the bottom. pH revealed that the lakes were circum-neutral with pH’s between 6.7 and 7.6.
From left to right: hammer coring device during sampling of thermokarst lake sediments, sediment core sampled for methane analysis and nucleic acids, and solid methanogenic medium with isolated colonies.
Methane concentration, methane oxidation rates, chlorophyll-a and bacterial density for the October 2011 study lakes.Mid-depth samples were collected for bulk methane levels and methane oxidation rate experiments, phytoplankton chlorophyll-a levels and bacterial abundance.
Bulk methane concentrations were several orders of magnitude in the sample from Sukok Seep, a site that also had the lowest chlorophyll-a levels. Rates of methane oxidation at the Sukok Seep site were more than 3 orders of magnitude greater than those in the other lakes samples indicating the presence of active methane oxidizing bacteria.
Our DNA samples were sent to a private sequencing company in Texas who pyrosequenced the 16S RNA genes. Unfortunately, a micro-fluidics error in their system produced data that were suspect. Remaining data from these samples was resequenced by the company and the data are awaiting alignment and quality control.
Bacterial productivity experiments, using 3H-leucine as a growth substrate, were conducted over temperatures ranging from 0 to 25 oC to determine the preferential growth temperature of the bacteria within each lake (ie, to determine if the bacteria in the study lakes were psychrophiles, mesophiles or thermophiles). The radioactivity in these samples has recently been determined and final rate calculations will take place shortly.
Biology
To study biological methane production from autochthonous organic matter, thermokarst lakes on the Northern Slope of Alaska were sampled in October 2011. Sediment cores were obtained from two sites at Sukok Lake (one at a methane seep, and the other near the middle of the lake) and one site in North of Walakpa. The potential for methane production in sediment horizons at three depths was evaluated over a 10 day period at 2 and 10 C. Methane production was only observed from North of Walakpa and one site at Sukok, with higher amounts of methane produced from the surface sediments at 10 C than the deeper sediments at 10 C, and less methane produced at 2 C at both sites. Methane production was also higher in North of Walakpa sediments than in Sukok sediments, and the temperature coefficient Q10 suggests that methane produced in North of Walakpa sediments responds to temperature increase, whereas the methane produced in Sukok is controlled by factors other than temperature. In situ methane concentrations were determined by sampling parallel cores in the field. Concentration of methane was higher in sediments from North of Walakpa than in sediments from Sukok, however the methane concentration increased with depth in Sukok as opposed to decreasing with depth like in North of Walakpa. To study the microbial community inhabiting these sediments and the members of the community who are responsible for methane production, frozen cores from these lakes were used for microbial community analyses. Community genomic DNA and total RNA were extracted, RNA was amplified, and cDNA from total RNA and from amplified RNA was used for Itag sequencing with primers for the 16S rRNA gene. By comparing the genomic DNA sequences to the cDNA sequences the active members of the community will be identified. Additionally, culture work has been continued in order to isolate methanogens from the sediments of the lakes for downstream work with psychrotolerant methanogens.
References
Dalton, J.B., R. Mogul, H.K. Kagawa, S.L. Chan, and C.S. Jamieson. 2003. Near-Infrared Detection of Potential Evidence for Microscopic Organisms on Europa, Astrobiology 3, 505-529.
Dalton, III, J.B., and K.M. Pitman. 2012. Low temperature optical constants of some hydrated sulfates relevant to planetary surfaces, J. Geophys. Res. 117:E09001, doi:10.1029/2011JE004036.
Dalton, III, J.B., D.P. Cruikshank and R.N. Clark. 2012a. Compositional analysis of Hyperion with Cassini VIMS, Icarus 220:752-776.
Dalton, III, J.B., J.H. Shirley and L.W. Kamp. 2012b. Europa’s icy bright plains and dark linea: Exogenic and endogenic contributions to composition and surface properties, J. Geophys. Res. – Planets, 117:E03003, doi:10.1029/2011JE003909.
Dalton, III, J.B., T. Cassidy, C. Paranicas, J.H. Shirley, L.M. Prockter, L.W. Kamp. 2012c. Exogenic controls on sulfuric acid hydrate production at the surface of Europa, Planet. Space Sci., in press.
Hapke, B., 1981. Bidirectional reflectance spectroscopy I. Theory, J. Geophys. Res. 86, 3039-3054.
Ivanov, M.L., L.M. Prockter and J.B. Dalton. 2011. Landforms of Europa and selection of landing sites, Adv. Space Res., 48:661-677, doi:10.1016/j.asr.2011.05.016.
Shirley, J.H., J.B. Dalton III, L.M. Prockter, L.W. Kamp. 2010. Europa’s ridged plains and smooth low albedo plains: Distinctive compositions and compositional gradients at the leading side – trailing side boundary, Icarus 210:358-384, doi:10.1016/j.icarus.2010.06.18.
Publications
-
Brad Dalton, J., Cruikshank, D. P., & Clark, R. N. (2012). Compositional analysis of Hyperion with the Cassini Visual and Infrared Mapping Spectrometer. Icarus, 220(2), 752–776. doi:10.1016/j.icarus.2012.05.003
-
Dalton, J. B., & Pitman, K. M. (2012). Low temperature optical constants of some hydrated sulfates relevant to planetary surfaces. Journal of Geophysical Research: Planets, 117(E9), n/a–n/a. doi:10.1029/2011je004036
-
Dalton, J. B., Cassidy, T., Paranicas, C., Shirley, J. H., Prockter, L. M., & Kamp, L. W. (2013). Exogenic controls on sulfuric acid hydrate production at the surface of Europa. Planetary and Space Science, 77, 45–63. doi:10.1016/j.pss.2012.05.013
-
Dalton, J. B., Shirley, J. H., & Kamp, L. W. (2012). Europa’s icy bright plains and dark linea: Exogenic and endogenic contributions to composition and surface properties. Journal of Geophysical Research: Planets, 117(E3), n/a–n/a. doi:10.1029/2011je003909
-
Hand, K. P., & Carlson, R. W. (2012). Laboratory spectroscopic analyses of electron irradiated alkanes and alkenes in solar system ices. Journal of Geophysical Research: Planets, 117(E3), n/a–n/a. doi:10.1029/2011je003888
-
Ivanov, M. A., Prockter, L. M., & Dalton, B. (2011). Landforms of Europa and selection of landing sites. Advances in Space Research, 48(4), 661–677. doi:10.1016/j.asr.2011.05.016
-
Korablev, O., Gerasimov, M., Brad Dalton, J., Hand, K., Lebreton, J-P., & Webster, C. (2011). Methods and measurements to assess physical and geochemical conditions at the surface of Europa. Advances in Space Research, 48(4), 702–717. doi:10.1016/j.asr.2010.12.010
-
Lazcano, A., & Hand, K. P. (2012). Astrobiology: Frontier or fiction. Nature, 488(7410), 160–161. doi:10.1038/488160a
-
Murray, A. E., Kenig, F., Fritsen, C. H., McKay, C. P., Cawley, K. M., Edwards, R., … Doran, P. T. (2012). Microbial life at -13 C in the brine of an ice-sealed Antarctic lake. Proceedings of the National Academy of Sciences, 109(50), 20626–20631. doi:10.1073/pnas.1208607109
-
Wilson, J. P., Grotzinger, J. P., Fischer, W. W., Hand, K. P., Jensen, S., Knoll, A. H., … Tice, M. M. (2012). DEEP-WATER INCISED VALLEY DEPOSITS AT THE EDIACARAN-CAMBRIAN BOUNDARY IN SOUTHERN NAMIBIA CONTAIN ABUNDANT TREPTICHNUS PEDUM. PALAIOS, 27(4), 252–273. doi:10.2110/palo.2011.p11-036r
- Priscu, J.C. & Hand, K.P. (2012). Microbial Habitability of Icy Worlds. Microbe, 7(4): 167-172.
-
PROJECT INVESTIGATORS:
-
PROJECT MEMBERS:
Heather Adams
Collaborator
Dan Berisford
Research Staff
Paula Matheus-Carnevali
Graduate Student
Megan Rohrssen
Graduate Student
Pamela Santibanez
Graduate Student
-
RELATED OBJECTIVES:
Objective 1.2
Indirect and direct astronomical observations of extrasolar habitable planets.
Objective 2.1
Mars exploration.
Objective 2.2
Outer Solar System exploration
Objective 4.1
Earth's early biosphere.
Objective 5.3
Biochemical adaptation to extreme environments
Objective 6.1
Effects of environmental changes on microbial ecosystems
Objective 6.2
Adaptation and evolution of life beyond Earth
Objective 7.1
Biosignatures to be sought in Solar System materials
Objective 7.2
Biosignatures to be sought in nearby planetary systems