2012 Annual Science Report
NASA Goddard Space Flight Center
Reporting | SEP 2011 – AUG 2012
Progress Report for the Cosmic Ice Laboratory
Project Summary
Scientists at the Cosmic Ice Laboratory with the Goddard Center for Astrobiology study the formation and stability of molecules under conditions found in outer space. During the past year, studies of amino-acid destruction were continued with one manuscript published and another in preparation. A project on the formation of sulfuric-acid hydrates was completed, and a new project involving thermal chemistry at Europa was started. Studies of frozen acetylene were performed and measurements of the infrared optical constants of frozen hydrocarbon molecules was begun. All of this work is part of the Comic Ice Laboratory’s continuing contributions toward understanding the chemistry of biologically-related molecules and chemical reactions in extraterrestrial environments.
Project Progress
Our research focuses on chemical reactions and physical properties of icy materials in extraterrestrial environments dominated by low-temperature and low-pressure conditions. Ionizing radiation sources are used to simulate space radiation conditions and to drive reactions. Infrared (IR) spectroscopy is used to monitor the chemistry in situ within a high-vacuum chamber. Our main tools for initiating chemistry are MeV and keV ion sources and UV lamps. With these tools we can investigate a wide variety of interstellar, circumstellar, and solar-system chemistry.
Over the past year we have continued our work on amino acids, moving away from molecular formation in interstellar ices to molecular destruction on planetary bodies. We also have followed up our earlier study of the radiation stability of sulfuric acid hydrates, as relate to Europa, by examining hydrate reformation in irradiated ices. Finally, we have extended our earlier work on reference mid-IR spectra, by addressing the specific case of solid-phase acetylene (C2H2), for application to Spitzer data.
Amino Acid Destruction by Radiolysis
In previous papers we have reported on the radiolytic and photolytic destruction of small molecules known to be in the interstellar medium (ISM). Recently, we have turned our attention to similar measurements on amino acids as a preliminary step toward examining even larger species. With NAI support, we have expanded substantially on our previous year’s work by measuring the radiolytic destruction rates of the amino acids glycine, alanine, and phenylalanine at three temperatures, both in the pure solid state and in the presence of water-ice. Our long-term goal is to obtain rate data for the radiolytic destruction of amino acids in various astronomical environments, such as on Mars, Titan, and the ISM. Figure 1, from our recent Icarus (2012) paper, compares the destruction of pure glycine and glycine trapped in H2O-ice. Surprisingly, such data from in-situ measurements below room temperature are otherwise unavailable. The figure clearly and quantitatively shows that the rate of glycine destruction is smaller when the glycine molecules are surrounded by H2O-ice. Figure 2 extends this conclusion to other two other amino acids, one aliphatic and one aromatic, at several concentrations and temperatures. The G value plotted is a standard quantity for measuring radiation-induced changes (large G ==> large change). Two conclusions that can be drawn are (1) that an amino acid’s destruction rate is smallest when H2O molecules are present to absorb part of the incident radiation’s energy and (2) that the destruction rates actually are smaller at higher temperatures. Extensions to more Mars-like conditions are underway in our laboratory.
Figure 1: Comparison of the decays of glycine and glycine in H2O-rich ice at 15 K. Data for the H2O mixture have been scaled such that the graph represents samples with the same initial number of glycine molecules.
Figure 2: Amino acid destruction in H2O-ice mixtures. The left-hand panel shows G(-M) at several temperatures for three amino acids, while the right-hand panel plots the same data as a function of molar mass. The larger G(-M) the greater the destruction rate
Radiolysis of Sulfuric-Acid Hydrates
Last year we completed a Europa-related study of the radiation products and destruction rates of sulfuric acid (H2SO4), sulfuric acid monohydrate (H2SO4 • H2O), and sulfuric acid tetrahydrate (H2SO4 • 4 H2O). We identified the main radiation products as H2O, SO2, (S2O3)x, H3O+, HSO4–, and SO42–. This past year we extended our sulfur studies to the post-irradiation recovery (reformation) of these forms of sulfuric acid. Figure 3, taken from our recent Icarus paper, shows that the recovery is almost 100% in this case. Similar results were obtained for H2SO4 and H2SO4 • H2O.
Figure 3: Infrared spectra of H2SO4 • 4 H2O (a) at 160 K, (b) after cooling to 86 K, (c) after irradiation with 1.8 × 1015 H+/cm2 at 86 K, and after warming to 160 K at 1 K/min for (d) 0 min, (e) 40 min, (f) 3.5 h, and (g) 14 h. Spectra were translated vertically for clarity. The tetrahydrate’s recovery is evident.
The astrobiological significance of this work is that these oxygen-rich species are not irreversibly destroyed by ionizing radiation. As irradiated sulfurous material is transported below Europa’s surface, it slowly will be warmed, reform sulfate ions, and be made available for sub-surface redox chemistry. We now are working to quantify the rates of sulfate-ion formation, especially from thermally-induced reactions between H2O2 and SO2. Extensions of this work to other strong oxidants (e.g., perchlorates, ozone) are planned. This work was done with support from the Goddard Center for Astrobiology as well as NASA’s Planetary Geology & Geophysics program.
Other Work
In the previous year, our paper on the IR optical constants of nitriles related to Titan was published. This past year we used the same procedures and equipment to record an extensive set of spectra for acetylene (C2H2) in four different ice matrices, and we are now extending this work to the generation of acetylene’s IR optical constants. Along these lines, in the past year we have measured, for the first time, the refractive index of hydrocarbons in the visible region, a necessary first step for determining their optical constants at IR wavelengths. Such data are needed for quantifying the abundances of organic molecules in the outer solar system, and perhaps elsewhere.
Figure 4: Infrared spectra of the ν5 vibration of C2H2 in H2O:C2H2 mixtures with ratios of (a) 1:1, (b) 5:1, (c) 10:1, and (d) 50:1 as a function of temperature. Spectra were normalized to 1.
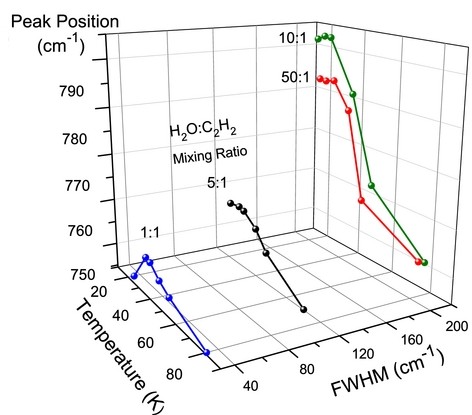
Figure 5: Variation of the peak position and FWHM of the ν5 vibration of solid C2H2 as a function of mixing ratio at different temperatures.
Publications
-
Gerakines, P. A., Hudson, R. L., Moore, M. H., & Bell, J-L. (2012). In situ measurements of the radiation stability of amino acids at 15–140K. Icarus, 220(2), 647–659. doi:10.1016/j.icarus.2012.06.001
-
Knez, C., Moore, M. H., Ferrante, R. F., & Hudson, R. L. (2012). LABORATORY IR STUDIES AND ASTROPHYSICAL IMPLICATIONS OF C 2 H 2 -CONTAINING BINARY ICES. The Astrophysical Journal, 748(2), 95. doi:10.1088/0004-637x/748/2/95
-
Loeffler, M. J., & Hudson, R. L. (2012). Thermal regeneration of sulfuric acid hydrates after irradiation. Icarus, 219(2), 561–566. doi:10.1016/j.icarus.2012.03.023
-
PROJECT INVESTIGATORS:
-
PROJECT MEMBERS:
Reggie Hudson
Co-Investigator
-
RELATED OBJECTIVES:
Objective 3.1
Sources of prebiotic materials and catalysts