2010 Annual Science Report
University of Wisconsin
Reporting | SEP 2009 – AUG 2010
Project 3C: Iron Isotope Biosignatures: Laboratory Studies and Modern Environments
Project Summary
Ancient rocks often carry chemical and isotopic signatures of ancient microbiological processes. However, fluids important in the generation of these signatures are lost upon lithification. Experimental studies in geochemical systems analogous to ancient rock precursors are therefore critical to gain insight into the biogeochemical processes responsible for generating unique chemical or isotopic compositions in ancient rocks. New laboratory studies were conducted to extend our recent work on Fe isotope fractionation during microbial dissimilatory iron reduction (DIR) in the presence of dissolved silica, which was likely abundant in Precambrian oceans. Iron isotope fractionation was investigated during microbial reduction of an amorphous iron oxide-silica coprecipitate in high-silica, low-sulfate artificial Archean seawater to determine if such conditions alter the extent of reduction, or the isotopic fractionations relative to those previously observed in simple systems. These new results show that, relative to simiple systems, significantly larger quantities of low-isotopically-light reduced iron were produced during reduction of the Fe-Si coprecipitate. These findings provide strong support for DIR as a mechanism for producing Fe isotope variations observed in Neoarchean and Paleoproterozoic marine sedimentary rocks.
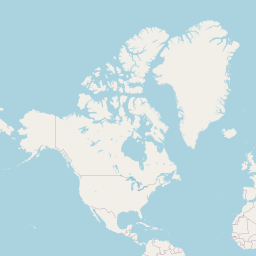
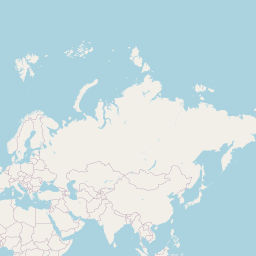
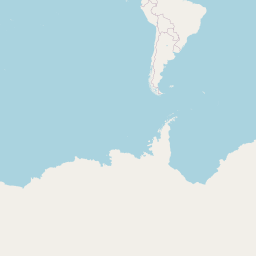
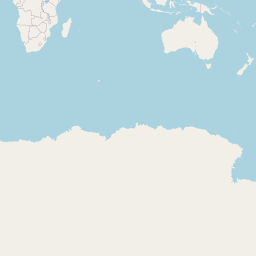
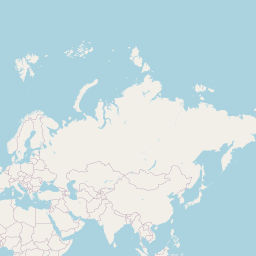
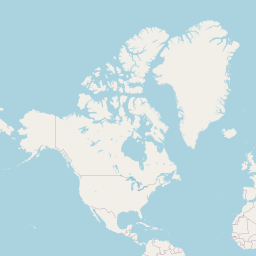
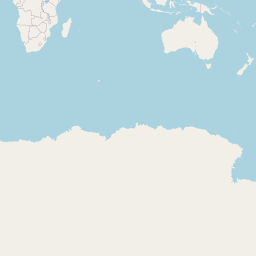
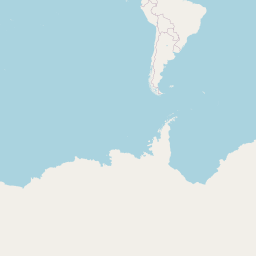
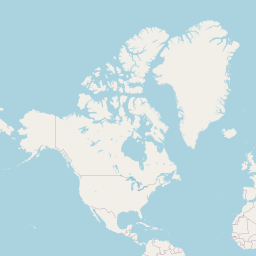
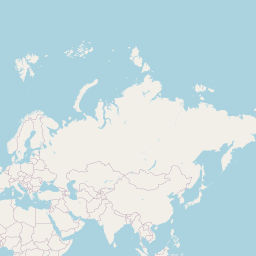
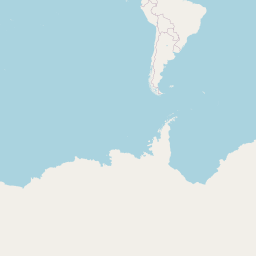
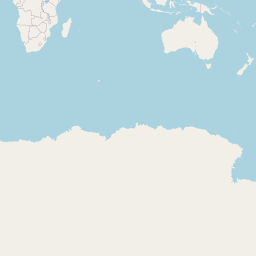
Project Progress
Microbial dissimilatory iron reduction (DIR) couples reduction of ferric oxides and hydroxides (referred to hereafter as Fe(III) oxides) to the oxidation of organic matter or H2. This metabolism is deeply rooted in the phylogenetic tree of life on Earth (Vargas et al., 1998 ; Lovley, 2004). DIR has been hypothesized to have played a role in Fe redox cycling in Precambrian marine environments (Canfield et al., 2006), potentially leaving behind geological (Nealson & Myers, 1990 ; Walker, 1984 ; Konhauser et al., 2002 ; Konhauser et al., 2005 ; Fischer & Knoll, 2009) and isotopic signatures (Beard et al., 2003 ; Johnson et al., 2008 ; Tangalos et al., 2010). In modern natural environments, an Fe isotope fingerprint appears to be associated with DIR in the form of low δ56Fe values for aqueous Fe(II) (Teutsch et al., 2009 ; Fehr et al., 2008 ; Severmann et al., 2006 ; Severmann et al., 2010 ; Tangalos et al., 2010). Previous experimental work on Fe isotope fractionation produced by DIR has used pure cultures and conditions analogous to freshwater systems (Crosby et al., 2007 ; Crosby et al., 2005 ; Johnson et al., 2005 ; Icopini et al., 2004), and to date only one study has looked at the effects of complex media such as the presence of dissolved silica (Wu et al., 2009). No work has investigated DIR-produced Fe isotope fractionation under conditions analogous to natural marine systems, especially those present in the Precambrian. Precambrian ocean chemistry was very different from that of modern oceans, due to the primitive atmosphere and, importantly, different controls on the silicon budget. In addition to low sulfate contents (e.g., Haicht et al., 2002), seawater in the Precambrian was likely saturated with respect to amorphous silica (Siever 1992 ; Maliva et al., 2005) because of the lack of silica-secreting organisms. It is possible that Fe(III) oxide-silica interactions impacted DIR in the Precambrian in ways not reflected in experiments using simple systems.
This study examined Fe isotope fractionations produced by DIR under conditions that simulated key aspects of Archean marine conditions, through use of an artificial seawater media that had a low sulfate concentration and high dissolved silica. In addition, the study used an amorphous Fe(III) oxide-silica coprecipitate designed to provide a better analog to Fe(III) oxide phases that likely existed in the silica-saturated Archean oceans relative to pure Fe(III) oxides.
Figure 1. The Fe-Si coprecipitate changed color from yellow-brown to dark green during microbial reduction (~ 80% reduction of Fe(III) content) (panel A); it remained essentially amorphous during reduction, as indicated by X-ray diffraction (panel B; note absence of peaks) and selected area electron diffraction (SAED) analysis on the TEM (panels C and D). The reduced material did, however, show evidence of localized zones of partial transformation to a primitive smectite-like phase (indicated by the white arrows) that were detectable by SAED (rings 1, 2, and 3, corresponding to 1.5, 2.5, and 4.5 Å diffraction lines, respectively). From Percak-Dennett et al. (2010).
These results demonstrated production of large quantities of Fe(II) in aqueous and solid phases that have distinct Fe isotope compositions, providing strong support for DIR as a highly efficient means for producing large quantities of isotopically-light Fe(II), as seen in
Figure 2. The results of this study are compared to those from prior studies of microbial reduction of hematite and goethite in the absence of Si (Crosby et al., 2007), microbial reduction of hematite in the presence and absence of Si (Wu et al., 2009), and microbial reduction of natural amorphous Fe(III) oxides (Tangalos et al., 2010). This study (data enclosed within box) produced a greater percent of reduction than observed in any experiments using synthetic electron acceptors, as well as lower d56FeFe(II) values for all Fe(II) components than previously identified. All d56Fe values were normalized to the isotopic composition of the starting material. From Percak-Dennett et al. (2010).
the Fe isotope record in Precambrian marine sedimentary rocks. Our results and interpretations also support and expand current concepts of how various diagenetic processes may have conspired to produce newly observed Fe isotope records both within ancient marine basins (Heimann et al., 2010) (Czaja et al., 2010) ancient marine basins.
Figure 3. The cartoon illustrates, in a series of steps, the potential impact of microbial Fe(III)-Si coprecipitate reduction on Fe isotope diagenesis. 1. Fe(III)-Si coprecipitate is deposited to the sediment column following coprecipitation of Fe(III) and Si in the water column. 2. The coprecipitate undergoes microbial reduction yielding inventories of Fe(II)aq, and solid-phase Fe(II) and Fe(III) (indicated here as being equivalent to the Fe pools extracted with 0.1 and 0.5 M HCl in our experiments). 3. Isotopically light (indicated by decreasing [downward arrow] d56Fe) Fe(II)aq produced by DIR is mobile and can be released into the water column, potentially leading to basin-scale segregation of light and heavy isotopes (Fe shuttle model of Severmann et al., 2006; 2008; see also Czaja et al., 2010). 4. Isotopically light slid-phase Fe(II) and isotopically heavy residual Fe(III) (indicated by increasing [upward arrow] d56Fe) produced during DIR are less mobile, and may undergo diagenesis (process 5) yielding small-scale heterogeneities in d56Fe of sediment Fe phases. 6. Solid-phase components may undergo remobilization during diagenesis leading to basin-scale isotopic heterogeneity. From Percak-Dennett et al. (2010).
References Cited
Beard BL, Johnson CM, Skulan JL, Nealson KH, Cox L, Sun H (2003) Application of Fe isotopes to tracing the geochemical and biological cycling of Fe. Chemical Geology, 195, 87-117.
Canfield DE, Rosing MT, Bjerrum C (2006) Early anaerobic metabolisms. Philosophical Transactions of the Royal Society B, 361, 1819-1834.
Crosby H, Johnson C, Roden E, Beard B (2005) Coupled Fe (II)-Fe(III) electron and atom exchange as a mechanism for Fe isotope fractionation during dissimilatory iron oxide reduction. Environmental Science and Technology, 39, 6698-6704.
Crosby HA, Roden EE, Johnson CM, Beard BL (2007) The mechanisms of iron isotope fractionation produced during dissimilatory Fe(III) reduction by Shewanella putrefaciens and Geobacter sulfurreducens. Geobiology, 5, 169-189.
Czaja AD, Johnson CM, Beard BL, Eigenbrode JL, Freeman KH, Yamaguchi KE (2010) Iron and carbon isotope evidence for ecosystem and environmental diversity in the similar to 2.7 to 2.5 Ga Hamersley Province, Western Australia. Earth and Planetary Science Letters, 292, 170-180.
Fehr MA, Andersson PS, Halenius U, Morth CM (2008) Iron isotope variations in Holocene sediments of the Gotland Deep, Baltic Sea. Geochimica et Cosmochimica Acta, 72, 807-826.
Fischer WW, Knoll AH (2009) An iron shuttle for deepwater silica in Late Archean and early Paleoproterozoic iron formation. Geological Society of America Bulletin, 121, 222-235.
Heimann A, Johnson CM, Beard BL, Valley JW, Roden EE, Spicuzza MJ, Beukes NJ (2010) Fe, C, and O isotope compositions of banded iron formation carbonates demonstrate a major role for dissimilatory iron reduction in ~2.5†Ga marine environments. Earth and Planetary Science Letters, 294, 8-18.
Icopini G, Anbar A, Ruebush S, Tien M, Brantley S (2004) Iron isotope fractionation during microbial reduction of iron: The importance of adsorption. Geology, 32, 205-208.
Johnson C, Beard B, Klein C, Beukes N, Roden E (2008) Iron isotopes constrain biologic and abiologic processes in banded iron formation genesis. Geochimica et Cosmochimica Acta, 72, 151-169.
Johnson C, Roden E, Welch S, Beard B (2005) Experimental constraints on Fe isotope fractionation during magnetite and Fe carbonate formation coupled to dissimilatory hydrous ferric oxide reduction. Geochimica et Cosmochimica Acta, 69, 963-993.
Konhauser K, Hamade T, Raiswell R, Morris R, Ferris F, Southam G, Canfield D (2002) Could bacteria have formed the Precambrian banded iron formations? Geology, 30, 1079-1082.
Konhauser K, Newman D, Kappler A (2005) The potential significance of microbial Fe (III) reduction during deposition of Precambrian banded iron formations. Geobiology, 3, 167-177.
Lovley DR (2004) Potential role of dissimilatory iron reduction in the early evolution of microbial respiration. In: Origins, Evolution and Biodiversity of Microbial Life (ed Seckbach J). Kluwer, Amsterdam, pp. 301-313.
Maliva RG, Knoll AH, Simonson BM (2005) Secular change in the Precambrian silica cycle: Insights from chert petrology. Geological Society of America Bulletin, 117, 835-845.
Nealson KH, Myers CR (1990) Iron Reduction by Bacteria – a Potential Role in the Genesis of Banded Iron Formations. American Journal of Science, 290A, 35-45.
Severmann S, Johnson CM, Beard BL, McManus J (2006) The effect of early diagenesis on the Fe isotope compositions of porewaters and authigenic minerals in continental margin sediments. Geochimica et Cosmochimica Acta, 70, 2006-2022.
Severmann S, McManus J, Berelson WM, Hammond DE (2010) The continental shelf benthic iron flux and its isotope composition. Geochimica et Cosmochimica Acta, 74, 3984-4004.
Siever R (1992) The silica cycle in the Precambrian. Geochimica et Cosmochima Acta, 56, 3265-3272.
Tangalos GE, Beard BL, Johnson CM, Alpers CN, Shelobolina ES, Xu H, Konishi H, Roden EE (2010) Microbial production of isotopically light iron(II) in a modern chemically precipitated sediment and implications for isotopic variations in ancient rocks. Geobiology, 1-12.
Teutsch N, Schmid M, Muller B, Halliday AN, Burgmann H, Wehrli B (2009) Large iron isotope fractionation at the oxic-anoxic boundary in Lake Nyos. Earth and Planetary Science Letters, 285, 52-60.
Vargas M, Kashefi K, Blunt-Harris EL, Lovley DR (1998) Microbiological evidence for Fe(III) reduction on early Earth. Nature, 395, 65-67.
Walker J (1984) Suboxic diagenesis in Banded Iron Formaitons. Nature, 309, 340-342.
Wu L, Beard BL, Roden EE, Johnson CM (2009) Influence of pH and dissolved Si on Fe isotope fractionation during dissimilatory microbial reduction of hematite. Geochimica et Cosmochimica Acta, 1-16.
Publications
-
Percak-Dennett, E. M., Beard, B. L., Xu, H., Konishi, H., Johnson, C. M., & Roden, E. E. (2011). Iron isotope fractionation during microbial dissimilatory iron oxide reduction in simulated Archaean seawater. Geobiology, 9(3), 205–220. doi:10.1111/j.1472-4669.2011.00277.x
-
PROJECT INVESTIGATORS:
-
PROJECT MEMBERS:
Brian Beard
Co-Investigator
Huifang Xu
Co-Investigator
Hiromi Konishi
Research Staff
Elizabeth Percak-Dennett
Graduate Student
-
RELATED OBJECTIVES:
Objective 2.1
Mars exploration.
Objective 4.1
Earth's early biosphere.
Objective 5.2
Co-evolution of microbial communities
Objective 6.1
Effects of environmental changes on microbial ecosystems
Objective 7.1
Biosignatures to be sought in Solar System materials
Objective 7.2
Biosignatures to be sought in nearby planetary systems