2010 Annual Science Report
NASA Jet Propulsion Laboratory - Titan
Reporting | SEP 2009 – AUG 2010
Task 2.1.1 Master Atmospheric Chemistry Simulation
Project Summary
The master atmospheric chemistry model will contribute to the understanding of the extent to which organic chemistry in atmospheric processes produces complex compounds.
Project Progress
Participant Karen Willacy, working with Principal Investigator Mark Allen, is developing a comprehensive model of the chemistry of organic compounds in Titan’s atmosphere to assess the extent to which organic chemistry develops in the atmosphere. This master model is an extension of the existing Caltech/JPL KINETICS model. The new reaction network is a combination of previous planetary atmosphere chemical networks, which comprise mainly reactions between neutral species, and interstellar networks of ion-molecule chemistry. The latter serve as a good starting point to simulate Titan ionospheric chemistry. The model also includes up-to-date descriptions of photodissociation and photoionization processes and treats the formation of the Titan haze. In addition it is possible to simulate thermochemical equilibrium, with rates of reverse reactions not explicitly included in the database, calculated by the microscopic reversibility method.
KINETICS (Allen et al. 1981) is a chemical/transport model developed for modeling planetary atmospheres. It has these characteristics:
- Coupled chemistry/dynamical model
- Eulerian: 1-D, 2-D and 3-D option; Lagrangian option
It has been previously used to model planetary atmospheres, molecular clouds, protostellar disks, and comet comae
The model inputs are: list of species and their abundances, reaction list, diffusion coefficients, physical conditions–density, temperature, pressure, boundary conditions, photo cross-sections, stellar/solar flux.
The model outputs abundances as a function of time and position.
The haze in Titan’s atmosphere provides the characteristic orange color of the atmosphere. It forms as a consequence of complex organic photochemistry. Lavvas et al. (2008) provided a microphysics description of the formation of the haze. This work provides a vertical profile of the haze constituents and abundances, which KINETICS will use to calculate the condensation area of the particles in the atmosphere. From this we can simulate the diffusion-limited condensation in the atmosphere.
Titan’s ionosphere is irradiated by UV photons, which cause photodissociation and photoionizaton. Cosmic rays also cause ionization. Ions may therefore play an important role in the chemistry of the upper atmosphere. We have included ion-molecule reactions based on interstellar chemistry databases. Additional neutral-neutral chemistry is included from recent Titan models and from combustion chemistry reaction databases. The new reactions come from
1. Interstellar databases:
a) UMIST RATE06 (www.udfa.net) (Woodall et al. 2007) Interstellar chemistry database, mainly focused on low temperature reactions (10 -100 K). Contains 4572 binary reactions, 420 species containing H, He, C, N, O, S, Si, Fe, Mg, Na,K, F,Cl. Largest species = H3C9N+, largest carbon chain = C10. Includes gas-grain interactions (freezeout and desorption of molecules onto grains) and grain surface reactions. See Figure 1.
b) OSU www.physics.ohio-state.edu/~eric/research_files/osu_01_2009
Interstellar chemistry database. Includes anions. Some high temperature chemistry.
c) Agundez et al. (2006)
Organic radical reactions
2. Recent Titan Atmosphere Models
a) Krasnopolsky (2009) b) Vuitton et al. (2009, 2007) c) Lavvas et al. (2008) d) Wilson & Atreya (2004)
3. Baulch et al. 2005
Combustion chemistry
The new version of KINETICS includes a total of 12490 reactions: 9621 two-body gas phase reactions of which 6636 are ion-molecule reactions, 354 three-body reactions, 1141 heterogenous reactions.
KINETICS requires photodissociation cross-sections for each molecule or atom as a function of wavelength (see Figure 2). These are then combined with the incident radiation field and relevant branching ratios to product photodissociation and photoionization rates at a given temperature. The KINETICS database has been updated to include new, high-resolution, temperature-dependent cross-sections taken from
- MPI-Mainz UV-VIS Spectral Atlas of Gaseous Molecules (www.atmosphere.mpg.de/enid/2295)
- HiTRAN database (cfa-ftp.harvard.edu/pub/HiTRAN2008)
- Science-Softcon database (www.science-softcon.de/uv-vis.html)
- SWRI database (amop.space.swri.edu)
Detailed chemical balance can be simulated by defining reverse reactions to complement forward reactions so that under appropriate conditions, thermodynamic chemical equilibrium can be computed. In every pair of detailed balance reactions, the rate expression for at least one reaction needs to be known from theoretical calculations or laboratory measurements. For reaction rates being computed by detailed balance equilibrium, KINETICS will use the rate information known for one reaction in the pair to compute the rates of the other reaction via the computation of the equilibrium rate constant
Keq = kf/kr
where Keq is the equilibrium constant, kf, kr are the forward and backward reaction rates constants, respectively.
References:
Agundez et al. (2006) A&A, 483, 831
Allen et al. (1981) JGR, 86, 3617
Baulch et al. (2005) J. Phys. Chem. Ref. Data, 34, 757
Burcat & Rusnic (2005) TAE 960, ANL-05/20, Argonne National Laboratory
Krasnopolsky (2009) Icarus, 201, 226
Lavvas et al. (2008) Planetary & Space Science, 56, 27
Vuitton et al. (2009) Planetary & Space Science, 57, 1558
Vuitton et al. (2007) Icarus, 191, 722
Wilson & Atreya (2004) JGR (Planets) 109, E06002
Woodall et al. (2007) A&AS, 466, 1197
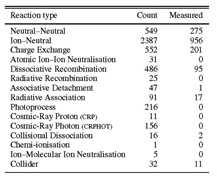
Figure 1. The number of each type of reaction included in RATE06, together with the many of the rates have been measured in the laboratory.
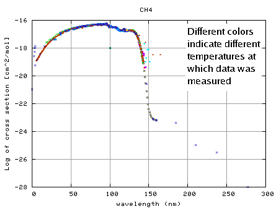
Figure 2. Log plot of the cross-section of methane, as a function of wavelength. Data taken at different temperatures are marked with different colors.
-
PROJECT INVESTIGATORS:
-
PROJECT MEMBERS:
Karen Willacy
Collaborator
-
RELATED OBJECTIVES:
Objective 1.1
Formation and evolution of habitable planets.
Objective 2.2
Outer Solar System exploration
Objective 3.1
Sources of prebiotic materials and catalysts
Objective 3.2
Origins and evolution of functional biomolecules
Objective 3.3
Origins of energy transduction