2010 Annual Science Report
NASA Jet Propulsion Laboratory - Icy Worlds
Reporting | SEP 2009 – AUG 2010
Habitability of Icy Worlds
Project Summary
Habitability of Icy Worlds investigates the habitability of liquid water environments in icy worlds, with a focus on what processes may give rise to life, what processes may sustain life, and what processes may deliver that life to the surface. Habitability of Icy Worlds investigation has three major objectives. Objective 1, Seafloor Processes, explores conditions that might be conducive to originating and supporting life in icy world interiors. Objective 2, Ocean Processes, investigates the formation of prebiotic cell membranes under simulated deep-ocean conditions, and Objective 3, Ice Shell Processes, investigates astrobiological aspects of ice shell evolution.
Project Progress
Objective 1: Seafloor Processes
This objective investigates conditions that might be conducive to originating and supporting life in icy world interiors. To enable prediction testing by this and other teams pursuing related questions, we will define biomarker production and look for ground-truth in analog terrestrial systems.
Project 1: Hydrothermal Reactor Experiment
Our main objective is to test the hypothesis that life can originate through a seafloor process that occurs at temperatures lower than those occurring in hydrothermal systems at mid-ocean ridges.
Our Year 2 objective is to analyzing aqueous, frozen, and precipitated products from isotopically-labeled reactants.
Progress: We have reproduced the kind of submarine exhalations predicted for wet/icy, rocky worlds (Geobiology, in press) and found at the Lost City hydrothermal site (calcium, silica, methane and minor formate in alkaline fluids with pH ≤12 at 100°C) but with the addition of bisulfide on reaction with iron sulfide (Astrobiology, in press and in preparation). We have shown that such fluids produce transition metal-bearing chimneys and compartments exhibiting regular external ornamentations to be expected in far-from-equilibrium conditions and indicative of physicochemical self-organization-ribs and rills enhanced by addition of short peptides, in preparation). We demonstrated detectability of methyl mercaptan and ethane using the Carbon Isotope Laser Spectrometer (CILS) and by extension, by the Curiosity Mars rover using the Tunable Laser Spectrometer (TLS) instrument on the Sample Acquisition Mission (SAM) package. CILS continues to be used in our hydrothermal experiments for the detection of methane, and now methyl mercaptan and ethane. In addition to a publication on the detectability of methyl mercaptan, seven papers have been published furthering the alkaline hydrothermal theory for the origin of life.
A new NAI/Caltech post doc, Dr Laurie Barge, and a new UCSB/JPL PhD student, Lauren Spencer-White, joined the hydrothermal team in July.
Fig. 1.1. Hydrothermal vent experiment 1 – left, sample six hours at rapid rate; right, sample six hours at slow rate.
Fig. 1.2. Environmental scanning electron micrograph taken of a cross section of an iron sulfide membrane (left0, and a zoomed in micrograph of spherical iron sulfide formations seen clinging to the inside of membrane. The iron:sulfur ratio of the membrane is ~6.5 (indicative of iron hydroxides) and the iron:sulfur ration of the spherical ations is ~1.2 (indicative of mackinawite, greigite, or pyrite with minor sulfide-poor impurities such as hydroxides). Note that this experiment was run under ambient laboratory conditions.
Project 2: Life outside the Habitability Zone Box
Our main objective is to test the hypothesis that a deep, cold, ocean can sustain life and to test the extent to which terrestrial life can adapt to such conditions, we culture facultative and obligate anaerobic microbes under simulated icy world conditions. This work will assume that an icy world ocean contains small amounts of organic compounds formed near regions of hydrothermal activity, abiotically as above, or as a byproduct of a long-lived – albeit low-productivity- ecosystem.
Our Year 2 Objectives include the followings: 1) Culture microorganisms under icy world conditions of T, P, and composition. 2) Investigate Fe, S, and C biogeochemical cycles mediated by microorganisms. 3) Study change in gene expression under icy world conditions Interface with other investigators regarding biosignatures produced in lab work
Progress: Damhnait Gleeson joined the project as a postdoctoral Research Associate in February of 2010 to carry out engineering tasks under the supervision of Xenia Amashukeli and to take responsibility for the cultivation work. Second year support was used to modify the pressure setup to allow cultivation of organisms under multiple sets of nutrient conditions, in addition to facilitating comparison of abiotic and biologically mediated reactions. This was achieved by sourcing and assembling multiple high-pressure cells in parallel (see Figure 1.3). Testing of the modified system is continues as of this writing.
Fig. 1.3. Modified pressure setup incorporating multiple high pressure cells in parallel, which can be used to evaluate microbiological response to multiple nutrient conditions with abiotic controls.
Eight bacterial strains (7 Shewanella and 1 Moritella), originally isolated from high pressure environments, such as deep ocean sediments, were selected and are undergoing evaluating of temperature ranges for growth. Capacity for sulfur metabolism in these organisms is also being tested. Shewanella putrefaciens in particular is previously known to reduce elemental sulfur and is demonstrating growth across the range of temperatures evaluated (4 – 25°C). Sulfur metabolisms to be investigated for these and other bacterial strains are shown in Figure 6. Summer Undergraduate Research Fellow (SURF) Joy Lu investigated the ability of the Clostridium bacterium, an obligate anaerobe, to form endospores under environmental conditions relevant to Europa.
Fig. 1.4. Sulfur redox metabolisms under investigation.
Protocols for characterization of biosignatures from cultivation experiments are being developed for Capillary Electrophoresis (CE), Gas Chromatography Mass Spectroscopy (GC-MS) and electron microscopy (SEM) to evaluate rates of reactions, biomolecular byproducts and biominerals generated during the course of experiments.
Objective 2: Ocean Processes
Investigates the formation of prebiotic cell membranes under simulated deep-ocean conditions, computationally assesses the distribution of material and heat through the ocean and ice shell, and seeks to improve relevant chemical literature through a set of high-pressure equation-of-state experiments.
- Project 1: Prebiotic Membranes in Deep Oceans
Research: Investigate the range of pH (if any) under which lipid bilayer vesicles form at high pressures in the presence of salts.
Year 2 Objectives: Set up fluorescence equipment / acquire calibration data Study the range of pH (if any) of bilayer vesicle formation in the presence of salts Analyze and publish results, interface with computational tasks
Progress: William Abbey and Summer student Roshan Nanu built on work by 2009 Summer student, Shelley Shaul, conducting a detailed study of fatty acid vesicle formation using the astrobiology lab’s Hitachi scanning fluorescence spectrometer. They demonstrated the use of 1-anilinonaphthalene-8-sulfonic acid (1,8-ANS) as a fluorescent indicator of vesicle formation (Figure 3) and confirmed the results of previous studies on vesicle formation in decanoic acid. They have begun performing similar studies at different temperatures.
Fig. 1.5. The stepwise increase in fluorescence intensity for different pH values is shown.
Fig. 1.6. Photomicrographs of decanoic acid samples at different pH values. At pH 7.81 and lower, distinct vesicular structures are visible. Crystalline structures at pH 7.81, assumed to indicate salt precipitation, are under investigation.
- Project 2: Mass and Heat Transport in Icy World Oceans
Research: Investigate the effects of salinity and turbulence on material and heat transport processes in icy world oceans, with implications for sustaining habitable “niches”.
Year 2 Objectives Evaluate role of turbulence in plume dynamics Evaluate role of precipitation in plume dynamics. Evaluate pressure and composition dependent melting effects on ice ceiling
Evaluate pressure and composition dependent melting effects on ice ceiling
Progress: Dr. Goodman and Mr. Lenferink installed a supercomputer system at Wheaton College and implemented a three-dimensional plume dynamics model, comparing the size, velocity, and temperature of turbulent plumes with theoretical predictions over a wide range of parameter space. Goodman also developed a single-column convection model, which will allow exploration the overall vertical structure of Europa’s ocean on a global scale. Drs. Goodman and Vance converted equation-of-state information garnered from FREZCHEM and laboratory experiments into a form useful for physical ocean modeling, and have successfully incorporated MgSO4 brine density data into the single-column model.
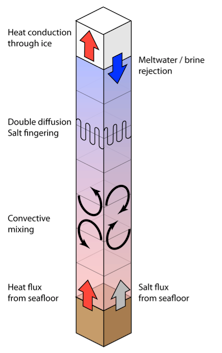
Fig. 1.7. Single-column convection model used to investigate vertical density structure of Europa’s ocean. Convective mixing is modeled as a vertical diffusive processs. Model includes heat and salt fluxes at top and bottom of ocean, full equation-of-state information for MgSO4 brines, and a parameterization for double-diffusive effects.
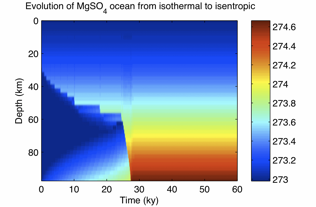
Fig. 1.8. Sample output from single-column convective model, using 0.3 molal MgSO4 brine. Initial condition is isothermal; thermally-driven convection from below and salinity-driven convection from above lead to an unstratified, isentropic ocean.
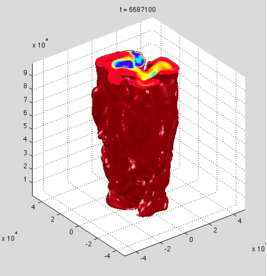
Fig. 1.9. 3-d plume dynamics model showing envelope of warm buoyant hydrothermal plume. Water depth 100 km, basal heat source 10 GW.
- Project 3: Icy World Ocean Chemistry
Research: Investigate the effects of low temperature and high pressure on the chemistry of icy world ocean solutions.
Year 2 Objectives: Obtain equations of state for aqueous ammonia under deep ocean pressures and temperatures. Incorporate existing and new data into chemical models such as FREZCHEM.
Progress: Co-I Vance is working during the months of October and November at the mineral physics lab at the University of Washington, in Seattle, to acquire sound velocities in aqueous ammonia, a possible analogue for an ocean in Saturn’s moon, Titan.
Objective 3: Ice Shell Processes
Investigates astrobiological aspects of ice shell evolution. Experimental work examines the creation of clathrate hydrates. Computational modeling of ice shell convection tracks the shuttling of these and other biorelevant materials between oceans and surfaces, and also examines the possibility of “sub-glacial” lakes as briny oases within the ice.
- Project 1: Clathrate Formation, Stability and Dynamics
Research: Investigate the stability of clathrate hydrate compounds under icy satellite conditions, thus providing information on their ability to store and bring material-possibly of biological origin-to the surface of icy satellites.
Year 2 Objectives: Investigate the stability of clathrates under icy world pressures and temperatures.
Progress: Reports from previous experiments on the stability of methane clathrates were published this year. These clathrates were found to be increasingly unstable with the addition of ammonia to aqueous solutions. A model for storage and cryovolcanic release of methane on Titan is discussed in this publication (Choukroun et al., 2010), which had just been accepted at the time of last year’s progress report.
Two-week-long experiments were carried out by Co-Is Choukroun and Grasset, and Erwan Le Menn (engineer) at the Laboratoire de Planetologie et Geodynamique, Universite de Nantes, in the H2O-CO2 and the H2O-CO2-MgSO4 systems. The loading procedure was modified from Choukroun et al. (2010a), with synthesis of clathrate hydrates before the runs in a separate high-pressure, low-temperature, vessel to load stoichiometric samples. Figure 1.10 shows the H2O-C2 phase diagram, with new dissociation data points that have been obtained by Choukroun (Ph.D. dissertation, 2007), and during the runs conducted this year. These data are consistent with those obtained by other authors in the low-pressure range, and allowed the exploration of the high-pressure stability of CO2 clathrate hydrates.
The experiments conducted in the H2O-CO2 system have shown a phase transition to a high-pressure phase of CO2 clathrate hydrates around 800 MPa. The structure of this phase, as could be seen optically and in the Raman spectral signature, presents strong similarities with the well-constrained high-pressure hexagonal phase of methane clathrate hydrates. This phase consistently transformed into ice VI and solid CO2 around 1200-1300 MPa, transition pressure that did not seem temperature-dependent over the range explored in the experiments. The reason for this dissociation under pressure remains to be explored. A publication that presents these results is in preparation (Choukroun et al., in prep.).
The test conducted on CO2 clathrates in a H2O-MgSO4 solution provided inconclusive results, primarily because the MgSO4 concentration was very low (<2%). Further tests are required to investigate further the influence of MgSO4 on the stability of clathrate hydrates at icy worlds conditions. In parallel to these experiments, co-I Choukroun has been very involved in publications dealing with the likely occurrence of clathrate hydrates in icy worlds, and the major effect they may have on their thermal evolution and geodynamic processes that shape their surface (Lunine et al., 2009; Sohl et al., in press; Fortes and Choukroun, in press; Choukroun et al., submitted).
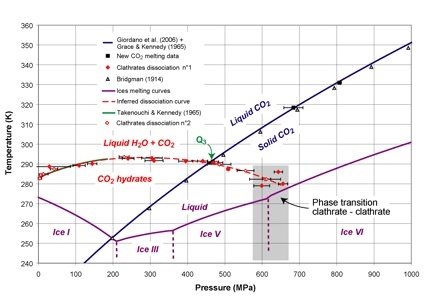
Fig. 1.10. Phase diagram in the H2O-CO2 system is shown.
- Project 2: Convection in Icy Lithospheres – simulating mass transport
Research: Trace mass transport in simulations of convecting in ice shells in order to address the transport of ocean materials, such as clathrate hydrates, salts, and biomolecules, from the interior to the surfaces of icy satellites.
Year 2 Objectives: Trace mass transport in computational models of convecting ice shells.
Progress: During year 2, we have conducted numerical simulations in 3D spherical coordinates and 3D Cartesian coordinates (thin shell) which allows us to run simulations on a (256×256×256) grid. We were interested in the scaling of the vertical velocity for realistic values of the viscosity. One key parameter which controls the stability of the thermal boundary layer is the activation energy. But the larger the activation energy, the more challenging the numerical simulation. During year 2, we have run 10 simulations with two different values of the viscosity at melting temperature (1013 and 1014 Pa.s) and 5 different values of the activation energy. The results are being processed. The velocities will allow us to address the question of how much time it takes for the particles embedded into the freezing ice at the base of the ice crust to reach the base of the conductive lid.
A second aspect of our numerical simulations is to investigate the horizontal stress applied by the convection process at the base of the conductive lid and to compare it with the yield stress necessary to break the ice lid. We have calculated these values for each of the 10 numerical simulations and data are being processed.
Project 3: Convection in Icy Lithospheres – multiphase model
Research: Develop a multi-phase ice shell model to assess role of liquids in ice shell habitability and transport.
Year 2 Objectives: In the second part of year 2, we will look at places where ice can melt and determine the amount of partial melting using the (P,T,composition) equations determined in project 1 (Choukroun and Grasset, 2010). Then we will adapt the multiphase code, which looks at the differentiation of liquid iron within silicate mantle to the case of percolation of liquid water in a ice matrix.
Publications
-
Choukroun, M., Grasset, O., Tobie, G., & Sotin, C. (2010). Stability of methane clathrate hydrates under pressure: Influence on outgassing processes of methane on Titan. Icarus, 205(2), 581–593. doi:10.1016/j.icarus.2009.08.011
-
Davies, A. G., Sotin, C., Matson, D. L., Castillo-Rogez, J., Johnson, T. V., Choukroun, M., & Baines, K. H. (2010). Atmospheric control of the cooling rate of impact melts and cryolavas on Titan’s surface. Icarus, 208(2), 887–895. doi:10.1016/j.icarus.2010.02.025
-
Fortes, A. D., & Choukroun, M. (2010). Phase Behaviour of Ices and Hydrates. Space Sci Rev, 153(1-4), 185–218. doi:10.1007/s11214-010-9633-3
-
Lorenz, R., & Sotin, C. (2010). The Moon That Would Be a Planet. Scientific American, 302(3), 36–43. doi:10.1038/scientificamerican0310-36
-
Nitschke, W., & Russell, M. J. (2009). Hydrothermal Focusing of Chemical and Chemiosmotic Energy, Supported by Delivery of Catalytic Fe, Ni, Mo/W, Co, S and Se, Forced Life to Emerge. Journal of Molecular Evolution, 69(5), 481–496. doi:10.1007/s00239-009-9289-3
-
Robuchon, G., Choblet, G., Tobie, G., Čadek, O., Sotin, C., & Grasset, O. (2010). Coupling of thermal evolution and despinning of early Iapetus. Icarus, 207(2), 959–971. doi:10.1016/j.icarus.2009.12.002
-
Sohl, F., Choukroun, M., Kargel, J., Kimura, J., Pappalardo, R., Vance, S., & Zolotov, M. (2010). Subsurface Water Oceans on Icy Satellites: Chemical Composition and Exchange Processes. Space Sci Rev, 153(1-4), 485–510. doi:10.1007/s11214-010-9646-y
-
Vance, S., Christensen, L. E., Webster, C. R., & Sung, K. (2011). Volatile organic sulfur compounds as biomarkers complementary to methane: Infrared absorption spectroscopy of CH3SH enables insitu measurements on Earth and Mars. Planetary and Space Science, 59(2-3), 299–303. doi:10.1016/j.pss.2010.08.023
- Choukroun, M., Kieffer, S., Lu, X. & Tobie, G. Clathrate Hydrates: implications for exchange processes in the outer Solar System [Book Chapter]. Solar System Ices.
- Gleeson, D.F., Amashukeli, X. & Pappalardo, R.T. (2010). An investigation into biologically mediated sulfur cycling under simulated Europa ocean conditions. DPS.
- Goodman, J.C. & Lenferink, E. (2009). Large-eddy Simulations of Europa Hydrothermal Plumes. Fall AGU Meeting. San Francisco, CA.
- Hussmann, H., Choblet, G., Lainey, V., Matson, D., Sotin, C. & Tobie, V.H.T. Internal Processes: Energy Sources and Heat Transport in Icy Satellites; Europlanet vol. on Icy Satellites.
- Jaumann, R., Kirk, R.L., Lorenz, R., Lopes, R.M.C., Stofan, E., Turtle, E.P., Keller, H.U., Wood, C.A., Sotin, C., Soderblom, L.A. & Tomasko, M.G. (2009). Geology and surface processes on Titan. In: Brown, R.H., Waite, H. & Dougherty, M. (Eds.). Titan from Cassini-Huygens. Springer-Verlag.
- Lunine, J.I., Choukroun, M., Stevenson, D.J. & Tobie, G. (2009). Origin and Evolution of Titan. In: Brown, R.H., Lebreton, J.P. & Waite, H. (Eds.). Titan from Cassini-Huygens. New York, NJ: Springer.
- Matson, D.L., Castillo-Rogez, J.C., S., G., S.C. & McKinnon, W.B. (2009). The Thermal Evolution and Internal Structure of Saturn’s Mid-Sized Icy Satellites. In: Dougherty, M. (Eds.). Saturn from Cassini-Huygens.
- Milner-White, E.J. & Russell, M.J. (2010). Polyphosphate-Peptide Synergy and the Organic Takeover at the Emergence of Life. Journal of Cosmology, 10: 3217-3229.
- Nitschke, W. & Russell, M.J. (2010). Just Like the Universe the Emergence of Life had High Enthalpy and Low Entropy Beginnings. Journal of Cosmology, 10: 3200-3216.
- Russell, M.J. & Hall, A.J. A hydrothermal source of energy and materials a the origin of life [Book Chapter]. Chemical Evolution II: From Origins of Life to Modern Society.
- Russell, M.J. & Kanik, I. (2010). Why Does Life Start, What Does It Do, Where Will It Be, And How Might We Find It? Journal of Cosmology, 5: 1008-1039.
- Schubert, G., Hussmann, H., Lainey, V., Matson, D., McKinnon, W., Sohl, F., Sotin, C., Tobie, G., Turrini, D. & Van Hoolst, T. (2010). Evolution of the Moons [Book Chapter]. Europlanet vol. on Icy Satellites.
- Vance, S. & Goodman, J. (2009). Oceanography of an Ice-Covered Moon [Book Chapter]. Europa. Arizona University Press.
- Yung, Y.L., Russell, M.J. & Parkinson, C.D. (2010). The search for life on Mars. Journal of Cosmology, 5: 1121-1130.
-
PROJECT INVESTIGATORS:
-
PROJECT MEMBERS:
Evan Abramson
Collaborator
John Baross
Collaborator
Lance Christensen
Collaborator
David Deamer
Collaborator
Richard Kidd
Collaborator
Robert Pappalardo
Collaborator
Mathieu Choukroun
Postdoc
Damhnait Gleeson
Postdoc
William Abbey
Research Staff
Randall Mielke
Research Staff
Lauren Spencer-White
Graduate Student
Roshan Nanu
Undergraduate Student
Laura Barge
Unspecified Role
-
RELATED OBJECTIVES:
Objective 1.1
Formation and evolution of habitable planets.
Objective 2.1
Mars exploration.
Objective 2.2
Outer Solar System exploration
Objective 3.1
Sources of prebiotic materials and catalysts
Objective 3.2
Origins and evolution of functional biomolecules
Objective 3.3
Origins of energy transduction
Objective 3.4
Origins of cellularity and protobiological systems
Objective 4.1
Earth's early biosphere.
Objective 5.1
Environment-dependent, molecular evolution in microorganisms
Objective 5.3
Biochemical adaptation to extreme environments
Objective 6.1
Effects of environmental changes on microbial ecosystems
Objective 6.2
Adaptation and evolution of life beyond Earth
Objective 7.1
Biosignatures to be sought in Solar System materials
Objective 7.2
Biosignatures to be sought in nearby planetary systems