2007 Annual Science Report
Marine Biological Laboratory
Reporting | JUL 2006 – JUN 2007
Iron Oxidation - Shaping the Past and Present Environments
Project Summary
Project Progress
This has been a big year for results, too many to detail thoroughly in this report (for further information and all presentation materials from our May meeting, see the “may meeting” folder at the ftp site above) Here I provide the highlights.
Results of nitrogen incubation experiments. We found that significant amounts of dinitrogen are produced in short term incubation ship-board incubation experiments. We are working towards improvement of the experimental protocol for 2007 so that we can trace the specific nitrogen transformation pathways, but in sum, experiments have shown significant amounts of denitrification occur on basalt surfaces.
Incubation for basalt-anammox bacteria. Previous results (Banning et al., 2006; Santeli et al 2006) indicate that anammox bacteria are present on the surfaces of basalt. We hypothesize that they are involved in the nitrogen cycle on basalt surfaces, and potentially play a role in Fe transformations as well. We initiated collaboration with Dr. Mike Jetten’s group in the Netherlands to attempt to cultivate these bacteria from Loihi basalts. The Bioreactor set is shown. We have a positive enrichment and are working towards identifying the organisms.
Rock biodiversity study. We are assessing the biodiversity of endolithic microbial communities on basalt at the Loihi seamount. MIT-WHOI Joint program student Erin Banning has extracted DNA and generated clone libraries for five rock samples (full-length 16S). Postdoctoral Beth Orcutt is now conducting phylogenetic analyses on these data.
We have been working on designing new Q-PCR probes for archaea (existing bacterial ones are reasonably efficient) based on archaeal sequences retrieved from Loihi (Augustine Dunn). We have also been optimizing (empirically with control cultures and different rock/mineral matrices) and calibrating our detection thresholds for extracting and amplifying DNA from rock using various protocols (Hongmei Wang). Presently, we are extracting DNA and applying bacterial Q-PCR primers, and will begin the same with new archaeal primers when that is complete.
A new hydrothermal field at Lohi: ‘Ula Nui In October on our cruise we discovered a new low-temperature hydrothermal field at the base of the Loihi Seamount that is unlike any previously described. We are preparing a Research Article for Science that will report the discovery of a novel low-temperature (0.2°C above ambient bottom-water temperatures) hydrothermal system at the base (5000 meter water depth) of the Loihi Seamount, Hawaii. This system was discovered on a cruise last fall as part of an Iron Microbial Observatory Project. The system is unlike any that has been described so far: 1) it occurs at significant distance from known active hydrothermal systems; 2) it’s exceptionally low temperatures, that despite the low temperature it is truly hydrothermal, not a seep; 3) it is very active biologically, and dominated by metal cycling bacteria; 4) it is a massive system, characterized by Iron and Manganese deposits in the form of mat fields that range from cm to many m in thickness and extend at about 100m x 100 m – this is all we could survey well. We could not always define the “edges” of the system.
The implication for this type of system contributing significantly to hydrothermal processes (chemical, bioloigcal) broadly in the ocean is impressive. This system also provides a genesis model for a type of geological deposit that has been recognized in the rock record for some time – Umbers. Umbers have typically have been interpreted as plume fall out but the stratigraphy of some of the massive Fe-Mn Umber deposits do not always match up.
Our collaborative work has also provided the following information:
Electron microscopy of Mariprofundus stalk ultrastructure and iron biominerals
We have analyzed the ultrastructure, chemistry, mineralogy and formation process of the Mariprofundus ferrooxydans stalk to better understand its role in iron oxidation and mineralization. Stalk ultrastructure, mineralogy and cell-stalk-mineral spatial relationships were observed using transmission electron microscopy (TEM). In collaboration with Sirine Fakra at the Advanced Light Source, Lawrence Berkeley National Laboratory, synchrotron-based scanning transmission x-ray microscopy (STXM) combined with near edge x-ray absorption fine structure (NEXAFS) spectroscopy was used to investigate elemental distribution and spatially-resolved chemical composition. Using these approaches we show that the stalks contain organic molecules that bind and encase ferric iron and transport it away from the cell before mineral precipitation and growth, thereby preventing cell entombment in iron oxyhydroxides. We have completed a draft of a manuscript describing this study. Below, we show representative data from this paper.
A key to this study was the preservation of fragile cell-stalk-mineral spatial relationships by careful sample handling. To accomplish this, TEM and STXM sample holders were inserted into a modified culture setup. Figure 1 shows a Mariprofundus cell attached to stalk, which is composed of discrete fibrils. High resolution imaging of ultrathin sectioned samples showed that stalk is initially mineralized initially by poorly crystalline FeOOH, and later coated with lepidocrocite. The juxtaposition of the poorly crystalline FeOOH within the stalk fibrils, and more crystalline lepidocrocite on the surface implies that organics in the fibrils retard mineral growth.


Synchrotron-based scanning transmission x-ray microscopy (STXM) was used to map the spatial distribution of iron, carbon, and nitrogen, and characterize the organic functional group chemistry of the stalk.
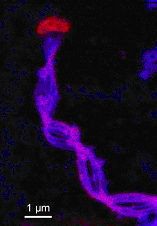
The element maps show that iron is localized on the stalk, whereas the cell is relatively free of iron. STXM-based NEXAFS spectra show that the stalk is composed of an organic polymer with carboxyl and other functional groups; there is ongoing work to better characterize the polymer composition. In sum, these data show that the stalk organics play an important role in the iron oxidation metabolism by binding ferric iron, retarding mineral growth and transporting it away from the cell in a manner that prevents entombment of the cell in iron oxyhydroxides.
We have also been studying microbial mat samples collected on a cruise to the Loihi seamount in October 2006, in order to compare the laboratory biominerals and morphologies with those from the environment. The mats contained abundant Mariprofundus-type stalks and a range of other morphologies, including one that was previously undescribed -branching tubes, rather than ribbon-like stalks.
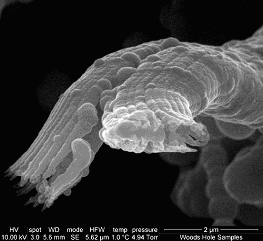
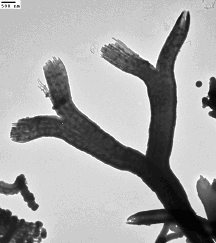
This is likely produced by a novel species of iron-oxidizer that has adopted a similar, but slightly different strategy of iron excretion and mineralization.
Time lapse light microscopy of live, stalk-forming cells
Because the stalk is mineralized as it forms, it is essentially instantaneously fossilized. Iron-containing filamentous morphologies have been observed in the rock record (e.g. Hofmann and Farmer 2000, Alt and Teagle 2003, Little et al. 2004); these are generally interpreted as microfossils and often compared to structures such as iron bacterial stalks. However, to be confident of the biogenicity of these putative microfossils and be able to interpret them, we need to know how the iron-oxidizing microbes make stalks and what the resulting stalk patterns (e.g. twisting, coiling, branching, overall direction) mean. To this end, we have been growing Mariprofundus in microcapillary slides and imaging cell growth and stalk formation by time lapse light microscopy. We are able to correlate cell motion with stalk morphology and measure rates of stalk growth (averages ~2μm/hr). Branching occurs when cells divide. The most notable result of these experiments is that it appears that the stalks align with the Fe/oxygen (opposing) gradients, and that the stalk-forming cells move towards regions of higher oxygen. This suggests that, on preservation, these stalks are not only evidence of biogenic activity, but they are a record of geochemical gradients. A review of putative iron microfossil images in the literature shows that these filaments/stalks are sometimes found to be parallel or subparallel. We suggest that a more careful analysis of these samples, comparing the details of filament morphologies and orientations with our observation, will allow for further interpretation of the conditions of deposition. If time permits, we will continue our microslide studies – this will involve altering and monitoring the conditions of the microslide cultures in order to better understand the chemical conditions of stalk formation and taxis.
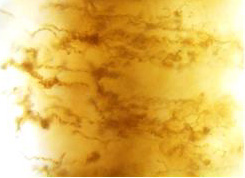
-
PROJECT INVESTIGATORS:
-
PROJECT MEMBERS:
Olivier Rouxel
Collaborator
Clara Chan
Postdoc
Beth Orcutt
Postdoc
Augustine Dunn
Research Staff
Erin Banning
Doctoral Student
Greg Horn
Doctoral Student
Cara Santelli
Doctoral Student
-
RELATED OBJECTIVES:
Objective 4.1
Earth's early biosphere
Objective 5.2
Co-evolution of microbial communities
Objective 5.3
Biochemical adaptation to extreme environments
Objective 6.1
Environmental changes and the cycling of elements by the biota, communities, and ecosystems
Objective 7.2
Biosignatures to be sought in nearby planetary systems