2013 Annual Science Report
Rensselaer Polytechnic Institute
Reporting | SEP 2012 – AUG 2013
Project 5: Vistas of Early Mars: In Preparation for Sample Return
Project Summary
To understand the history of life in the solar system requires knowledge of how hydrous minerals form on planetary surfaces, and the role minerals may play in the development of potential life forms. The minerals hematite and jarosite have been identified on Mars and presented as in situ evidence for aqueous activity. This project seeks to understand (i) the conditions required for jarosite and hematite formation and preservation on planetary surfaces, and (ii) the conditions under which their “radiometric clocks” can be reset (e.g., during changes in environmental conditions such as temperature). By investigating the kinetics of noble gases in minerals, known to occur on Mars and Earth, we will be prepared to analyze and properly interpret ages measured on samples from future Mars sample return missions.
Project Progress
5. Vistas of Early Mars: In Preparation for Sample Return (Baldwin, Das, Gombosi, Watson, Delano)
5.1 Background
The search for habitable environments on Mars and elsewhere in our solar system requires an understanding of the role minerals may have played in the development of potential life forms and when hydrous minerals formed on planetary surfaces. We are evaluating the potential of noble gases in Martian minerals to record the timing of surface and atmospheric conditions that can be used to characterize past habitable environments on Mars. By investigating the kinetics of noble gases in minerals, known to occur on Earth, Mars and the Moon, we aim to understand how common minerals found in our solar system can be used to determine the timing and rates of processes relevant to astrobiology. Results are aimed at developing protocols to analyze and properly interpret ages measured on samples from future Mars sample return missions.
5.2 Progress Report
Building upon results of Ar diffusion experiments of jarosite (Kula and Baldwin 2011), and models of Ar retention in jarosite and He in hematite, we determined conditions under which these mineral’s “radiometric clocks” can be reset, and developed a sampling strategy to determine the timing, duration and rates of water saturation in rocks on the surface of Mars (Kula and Baldwin 2012). On Mars, jarosite is thought to have formed during the acidic, water limited, sulphate-forming era (e.g., Hesperian). In the past year we have focused on the development of noble gas techniques that can be applied to minerals that also formed when warmer, wet conditions were prevalent on Mars (e.g., Noachian). The goal is to develop strategies to fully extract information from the rock record about environmental transitions that can then be used to assess the potential role of minerals in the development of life. We are not only interested in determining the timing of mineral formation, conditions required to preserve this age information, but also assessing the potential for trapped non-radiogenic noble gases to provide insight into the composition of the atmosphere at the time of mineral formation. The following outlines technique development, and noble gas experiments in progress, on key minerals to determine their ages and non-radiogenic isotopic compositions.
Technique development for coupled neon and argon analysis of minerals
Noble gas procedures were developed, using a Janis high-temperature closed cycle cryogenic cold trap system, to separate Ne from Ar prior to analysis of noble gases extracted from minerals during step heat experiments. This separation minimizes the interferences on 20Ne and 22Ne from doubly charged 40Ar and 44CO2 respectively. Figure 1 gives an example of neon isotopic results on air standards delivered from a calibrated pipette system. Using the protocol developed, in a pilot study of terrestrial micas and pyroxenes that formed at mantle depths, we have demonstrated these minerals contain trapped atmospheric Ar and Ne, indicating that atmospheric noble gases on Earth can be recycled to mantle depths on Earth (Baldwin & Das 2013). Survival of trapped atmospheric gases in minerals can be used to understand ancient atmospheres and their evolution through time.
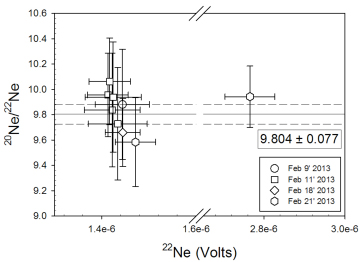
40Ar/39Ar dating of plagioclase from lunar regolith
Plagioclase, a framework silicate within the feldspar group of minerals, has been identified on Mars, on the Moon, and is a major constituent of the Earth’s crust. Plagioclase feldspar has been found in Meridiani planum on Mars (Glotch et al. 2006) and in Martian meteorites (McSween and Treiman 1998). The thermal emission spectrometer on Mars Global surveyor suggests that plagioclase is present in the Martian crust with an average composition of ~ An60 (Milam et al. 2010). We are testing the strategy of whether 40Ar/39Ar ages on individual feldspars from a sieved sample of regolith (e.g., basaltic eolian sands) can be used to infer the regional history of volcanism and impact events. Noble gas experiments are underway on lunar plagioclase crystals (An90-98) from Apollo 17 regolith sample (78461), derived from a region of the Moon whose history is known from over 40 years of rock analyses. Following irradiation in the (CLICIT) facility at Oregon State University, individual plagioclase grains (ranging from 0.1 to 0.7 micrograms) were outgassed using a CO2 laser. Argon isotopes were measured and 40Ar/39Ar ages obtained on 11 grains ranged from 3.5 to 4.1 Ga, similar to earlier reported values. A 36Ar/40Ar 1 was used to correct for the non-radiogenic 40Ar trapped component to estimate the total radiogenic 40Ar in the samples. However, it is observed that 40Ar/39Ar ages are correlated with the measured ratio of 36Ar/40Ar suggesting improper correction for the non-radiogenic component. One plagioclase sample (SU19E11;plag#14) yielded a 36Ar/40Ar ~ 1.5 indicating the presence of solar argon in this sample. These observations suggest that it is necessary to characterize the trapped non-radiogenic component present in these lunar samples. We are currently engaged in measuring Ar and Ne in natural (i.e., unirradiated) lunar plagioclase separated from same population of grains extracted from the lunar regolith. Results will be used in developing sampling strategies for a Mars sample return mission.
Quartz crystallization on Early Earth
We are also collaborating with Watson’s group to develop techniques that will allow scientists to obtain information about the conditions (P-T-time-deformation) on early Earth (3.0 to 4.5 billion years ago) by extracting noble gases from minerals that have survived since that time. We are targeting quartz from metamorphosed conglomerate in the Jack Hills of the Narryer Gneiss Terrane of Western Australia, the source of the oldest minerals (i.e., detrital zircons) known on Earth. Our objective is to develop techniques to date quartz and determine the non-radiogenic trapped noble gas compositions in quartz. The trace amounts of K (< 3 ppm) in quartz render these experiments particularly challenging. Also, the quartz samples are known to have inclusions (<2-3 µm) of K-bearing fuchsite (a Cr–rich variety of muscovite). Therefore one must be sure to avoid potential contributions from fuchsite in order to date quartz precisely. Thick sections of quartz cobbles and hydrothermal quartz crystals were obtained from the conglomerate. Prior to irradiation, one surface of these sections was polished and chemically characterized using the electron probe facility at Renesselaer Polytechnic Institute (RPI). X-ray maps for K were obtained (i.e., to identify fuchsite). It was found that fuchsite is generally restricted to quartz grain boundaries. Samples were irradiated for 100 hours in the Cadmium-Lined In-Core Irradiation Tube (CLICIT) facility at Oregon State University. Spot analyses on polished, well-characterized irradiated quartz thick sections are planned utilizing an excimer laser so that we can avoid potential interference from the fuchsite grains.
References:
Glotch, T. D., J. L. Bandfield, P. R. Christensen, W. M. Calvin, S. M. McLennan, B. C. Clark, A. D. Rogers, and S. W. Squyres (2006), Mineralogy of the light-toned outcrop at Meridiani Planum as seen by the Miniature Thermal Emission Spectrometer and implications for its formation, J. Geophys. Res., 111, E12S03, doi:10.1029/2005JE002672.
Kula, J., Baldwin. S.L., 2011, Jarosite, argon diffusion, and dating aqueous mineralization on Earth and Mars, Earth and Planetary Science Letters 310, 314–318.
Kula, J., Baldwin, S.L., 2012, On hematite as a target for dating aqueous conditions on Mars, Planetary and Space Science, Volume 67, Issue 1, p. 101-108. doi: 10.1016/j.pss.2012.03.005.
McSween H. Y., and Treiman A H., (1998) Martian meteorites, Planetary Materials: Reviews in mineralogy, Vol. 36 pp. 6-01,6-54.
Milam, K. A., H. Y. McSween Jr., J. Moersch, and P. R. Christensen (2010), Distribution and variation of plagioclase compositions on Mars, J. Geophys. Res., 115, E09004, doi:10.1029/2009JE003495.
Ozima M., and Podosek F. A. (2001) Noble Gas Geochemistry, 2nd edition, Cambridge University Press, Cambridge.
Publications
- Gombosi, D.J., Baldwin, S.L., Watson, E.B., Swindle, T.D., Delano, J.W. & Roberge, W.G. (2013). Argon Diffusion in Apollo 16 Impact Glass Spherules: Implications for 40Ar/39Ar Dating of Lunar Impact Events. Geochimicha et Cosmochimica Acta.
-
PROJECT INVESTIGATORS:
-
PROJECT MEMBERS:
John Delano
Co-Investigator
Bruce Watson
Co-Investigator
Jayesh Das
Collaborator
David Gombosi
Collaborator
Joseph Kula
Collaborator
-
RELATED OBJECTIVES:
Objective 1.1
Formation and evolution of habitable planets.
Objective 2.1
Mars exploration.
Objective 7.1
Biosignatures to be sought in Solar System materials